All published articles of this journal are available on ScienceDirect.
Acid Etching and Plasma Sterilization Fail to Improve Osseointegration of Grit Blasted Titanium Implants
Abstract
Interaction between implant surface and surrounding bone influences implant fixation. We attempted to improve the bone-implant interaction by 1) adding surface micro scale topography by acid etching, and 2) removing surface-adherent pro-inflammatory agents by plasma cleaning. Implant fixation was evaluated by implant osseointegration and biomechanical fixation.
The study consisted of two paired animal sub-studies where 10 skeletally mature Labrador dogs were used. Grit blasted titanium alloy implants were inserted press fit in each proximal tibia. In the first study grit blasted implants were compared with acid etched grit blasted implants. In the second study grit blasted implants were compared with acid etched grit blasted implants that were further treated with plasma sterilization. Implant performance was evaluated by histomorphometrical investigation (tissue-to-implant contact, peri-implant tissue density) and mechanical push-out testing after four weeks observation time.
Neither acid etching nor plasma sterilization of the grit blasted implants enhanced osseointegration or mechanical fixation in this press-fit canine implant model in a statistically significant manner.
INTRODUCTION
In the western world, more than 2% of the population over 60 years receive a total hip replacement [1] and each year one million hip replacements are inserted worldwide. Implant failure due to aseptic loosening is a serious, painful and potentially invalidating complication. 1 to 5% of all uncemented hip implants fail within fifteen years of implantation [2].
Rapid initial fixation of the implant is imperative to ensure long-term implant survival [3]. If the implant is not stable, micro motion between the implant and the surrounding bone will increase the risk of fibrous encapsulation of the implant [4, 5], which inhibits bone ingrowth and thus increases the risk of loosening of the implant.
The macro-scale porosity of the implant surface plays an important role in osseointegration of the implant [6]. Furthermore, in recent years the concept of using micro-scale topography to stimulate bone forming osteoblasts has been investigated by several different groups [7-9]. Applying micro-scale topographical changes onto clinically rough surfaces has, however, proven difficult.
Etching of titanium implants enables the application of micro-topographical changes onto macro-scale porosities [10]. In vivo studies have shown enhanced osseointegration of acid etched implants compared to non-etched controls [11-13]. Furthermore, etching a grit blasted surface eliminates the inherent problem of ceramic particle residues thereby reducing the particle load in the peri-implant space.
In addition, superior implant biocompatibility may further improve osseointegration. Endotoxins are molecules from the cell walls of bacteria, that induce a massive inflammatory response from macrophages leading to fibrous capsule formation [14]. Implants surrounded by a fibrous capsule have an increased probability of loosening, compared to prostheses that are directly anchored in bone [15]. Endotoxins have previously been found extensively on the surface of commercially available implants [16]. Consequently, increasing the biocompatibility of implants osseointegration and reducing the probability of implant loosening by removal or inactivation of the endotoxins from the surface of the implants [14] by plasma sterilization appear promising [17, 18].
We hypothesized that acid etching improves early osseointegration and implant fixation of grit blasted titanium implants as evidenced by improved biomechanical implant fixation, increased formation of new bone, and decreased presence of fibrous tissue. Furthermore, we hypothesized that removing the bio-burden by plasma sterilization of the implants would improve these parameters additionally.
MATERIALS AND METHODOLOGY
Design
The study consisted of two separate sub-studies, both designed as paired animal studies. A total of ten skeletally mature Labrador dogs were used. Mean body weight was 34 kg [range 25 kg: 39 kg]. Mean age was 18 months [range 14-23 months]. Each dog had two implants inserted medially in the proximal part of the tibia. Each dog had four implants, so a total of 40 implants were used. The implants were inserted press-fit in cortico-cancellous bone. In the upper tibia site, we investigated the effect of surface etching on grit blasted implants. At the more distal tibia site we investigated the effect of combining surface etching and plasma sterilization on grit blasted implants. Due to denser bone quality, the upper tibia surgical site offered a better biomechanical implant fixation compared to the lower site (p=0.0098). Hence, no cross comparisons between the two sub-studies was performed. The observation time was four weeks (Fig. 1).
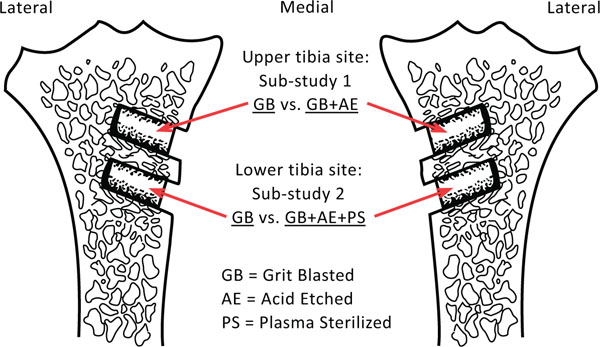
Schematic drawing of the placement of the implant pairs in the right and left tibia.
Animals and Surgical Procedure
Under general anesthesia, using sterile technique, the proximal part of tibia was exposed through an anteromedial extraarticular approach. Two Kirschner (K) wires were inserted perpendicular to the surface with 15.0 mm in between. The most proximal K wire was inserted with the implant centre 15 mm from the tibia plateau. The K-wires guided the 5.5 mm cannulated drill creating 11.0-mm-deep holes. Drilling was performed at two rotations per second to prevent thermal trauma to the bone. All bone debris and soft tissue was removed from the drill hole before the implant was inserted (press fit). Finally, the soft tissue was closed in layers. Pre-and postoperatively, the dogs were given one dose of Cefuroxim, 1.5 g intravenously, as antibiotic prophylaxis. A fentanyl transdermal patch (75 μg/h) lasting three days was given as postoperative analgesic treatment. The dogs were allowed full weight bearing postoperatively. The dogs were bred for scientific purposes, and the study was approved and monitored by the Danish Animal Research Committee (Fig. 2).
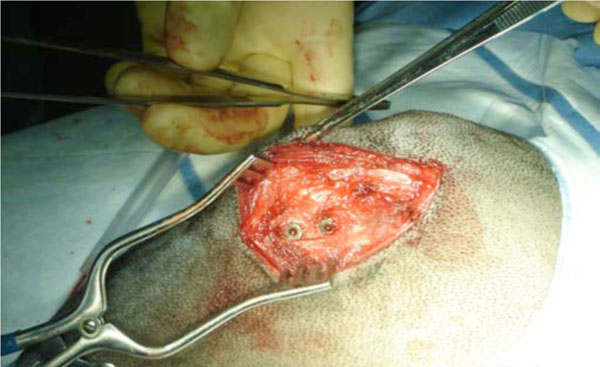
Peri-operative picture showing the two implants inserted medially in the proximal part of the right tibia.
The Implants
The aluminum oxide (Al2O3) grit blasted implants (L = 10 mm, Ø = 6 mm) were made out of Ti-6Al-4V alloy (Depuy Inc., Warsaw, IN, USA). The implants had a central threaded hole which in combination with a threaded rod made it possible to handle and insert the implants without touching and thereby potentially polluting the implant surface. The implants were prepared as follows.
Acid etching: Acid etching was done at room temperature in an acidified NaF solution for six minutes. The implants were then rinsed with a 1% Alconox (Alconox, Inc., White Plains, NY, USA) and 2% Liquinox (Alconox, Inc., White Plains, NY, USA) detergent at 45ºC for 30 minutes. The implants were then rinsed three times in 15 minute cycles with RO-H2O at 45ºC. Finally the implants were dried at 60ºC.
Plasma sterilization: The plasma sterilization with pure O2 gas was performed in a 500 Watt plasma chamber (7200 RF Plasma Processing System; PVA TePla America, Inc.) with a gas flow rate of 250 standard cubic centimetres per minute (sccm) and a chamber pressure of 300 mTorr (40 x 10-5 bar). The cycle time was 30 minutes.
Passivation/gamma sterilization: After standard cleaning in the lab passivation of the implants was carried out using the validated (ASTM A967-05) manufacturing passivation process. Parts were passivated using 35% Nitric Acid at 60°C. The cleaning process involved a series of temperature controlled water baths. The samples were dried and packaged by trained employees in the clean room. Parts were then Gamma Sterilized using the same processing plant used for standard DePuy (Depuy Inc., Warsaw, IN, USA) Implants. All of these processes are validated manufacturing processes used for actual DePuy (Depuy Inc., Warsaw, IN, USA) Orthopaedic Implants.
The implants were packaged individually in glass vials with screw lids. The glass vials were packaged in two layers of sterile packaging and one layer of unsterile packaging (a cardboard box).
The implants were attached to the lid with a threaded rod screwed into the hole in the implant. This way the implants did not touch the inside of the glass vial and could be handled and inserted using the rod, without risk of polluting it with endotoxins from sterile gloves or instruments (Fig. 3).
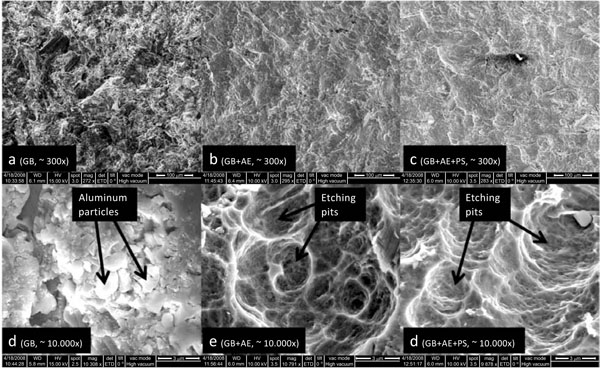
SEM pictures of the implant surfaces: In picture d, crystals of aluminum oxide (Al2O3 particles) from the grit blasting are clearly visible. Pictures e and f show the pits created by the etching process and the pictures indicate that the majority of the particles has been removed by the etching process.
Specimen Preparation
After four weeks of observation the animals were sacrificed with an overdose of hyper saturated barbiturate and the proximal part of tibia was excised and cleaned and thereafter stored at –20°C. The outermost 0.5 mm of the implant-bone specimen interphase was cut off and discarded. The rest of the implant with surrounding bone was divided into two sections perpendicular to the long axis of the implant with a water-cooled diamond band saw (Exact Apparatebau, Nordenstedt, Germany). The outermost section was cut to a thickness of 3.5 mm and stored at –20°C pending mechanical testing [19]. The innermost part 6.0 mm was prepared for histomorphometry. The specimens were dehydrated in graded ethanol (70-100%) containing basic fucsin, and embedded in methylmetacrylate (Technovit 7200 VCL; Exact Apparatbau, Nordenstedt, Germany). Four vertical, uniform, random sections were cut with a hard-tissue microtome (KDG-95; MeProTech, Heerhugowaard, The Netherlands) around the center part of each implant as described by Overgaard et al. [20]. Before making the sections, each cylindrical implant was rotated randomly around its longitudinal axis. The sections were cut parallel to this axis. The 40-μm-thick sections were counterstained with 2% light green (BDH Laboratory Supplies, Poole, UK) and then mounted on glass [21]. This preparation provides red staining of non-calcified tissue and green staining of calcified tissues such as woven and lamellar bone.
Histomorphometrical Evaluation
Blinded histomorphometric analysis was done using a stereological software program (C.A.S.T.-grid Olympus Denmark A/S, Ballerup, Denmark). Fields of vision from a light microscope were captured on a computer monitor and a user-specified grid was superimposed on the microscopic fields. Four vertical sections representative of each implant were analyzed and cumulated [20].
With the aid of the software, we defined two zones in the sections. Zone 1 stretches from the implant surface and 500 μm outwards into the surrounding tissue, and Zone 2 was defined starting 500 μm from the same implant outer surface line and extending another 500 μm outwards into the surrounding tissue.
In both zone 1 and 2 tissue volume fractions were quantified by point counting [22]. On the implant surface, line intercept-technique with sine-weighted lines was used to estimate the bone-implant contact [23].
Intra-observer variation was determined as coefficients of variation on double measurements on four randomly selected implants. All the coefficients were well within the expected range; however, defining small amounts of new bone on the implant surface and discriminating between new and lamellar bone close to the implant proved troublesome (new bone in zone 1 [24%], lamellar bone on the surface [52%]) (Fig. 4).
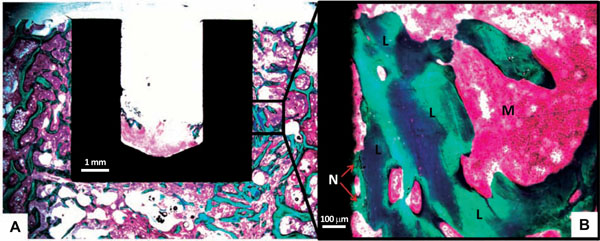
(A) Picture showing a histological cross section used for Histomorphometry. The close-up picture (B) shows Lamellar Bone (L) and New Bone (N) in contact with the implant surface. Marrow is marked (M).
Mechanical Testing
The thawed implants were tested for failure by axial push-out test on a MTS Bionics Test Machine (MTS, Eden Prairie, MN, USA). The specimens were placed on a metal support jig with a 7.4 mm diameter central opening. The implant was centralized over the opening, assuring a 0.7 mm distance between the implant and the support jig. The direction of loading was from the cortical surface inward. A preload of 2 N was applied to standardize contact conditions before initiating loading. We used a displacement rate of 5 mm/min with a 2,5 kN load cell. Load and deformation were registered by a personal computer. Each specimen length and diameter was measured with a micrometer screw gauge and used to normalize push-out parameters [24]. Ultimate shear strength (MPa) was determined from the maximal force applied until failure of the bone-implant interface. Apparent stiffness (MPa/mm) was obtained from the slope of the linear section of the curve. Energy absorption (kJ/m2) was calculated from the area beneath the curve until failure.
Statistics
Histomorphometrical as well as mechanical data followed a normal distribution and a paired t-test was used to distinguish between groups. The data were presented as means with standard deviation (SD). Differences between means were considered statistically significant for p-values less than 0.05.
RESULTS
Surgery
All dogs were fully weight bearing within three days of surgery. No postoperative complications were seen, and no dogs were excluded during the observation period. No signs of infection were seen during the harvesting of the specimens.
Histomorphometrical Results (Tables 1 and 2)
Sub-Study 1 (Upper Site)
Tissue Area and Volume Fractions
Surface (0 µm): Tissue Area Fraction in Percent
Zone 1 (0-500 µm): Tissue Volume Fraction in Percent
Zone 2 (500-1000 µm): Tissue Volume Fraction in Percent
Sub-Study 1 | New Bone | Lamellar Bone |
---|---|---|
Surface | Mean (SD) | Mean (SD) |
GB | 48.8 (8.4) | 6.0 (4.2) |
GB+AE | 47.4 (11.3) | 8.2 (8.3) |
P-values | 0.786 | 0.443 |
Zone 1 | Mean (SD) | Mean (SD) |
GB | 28.9 (5.0) | 16.7 (7.2) |
GB+AE | 26.9 (7.9) | 17.3 (9.0) |
P-values | 0.540 | 0.868 |
Zone 2 | Mean (SD) | Mean (SD) |
GB | 18.2 (6.2) | 21.7 (3.8) |
GB+AE | 19.0 (7.9) | 21.6 (10.0) |
P-values | 0.826 | 0.972 |
Sub-Study 2 (lower site).
Tissue Area and Volume Fractions
Surface (0 µm): Tissue Area Fraction in Percent
Zone 1 (0-500 µm): Tissue Volume Fraction in Percent
Zone 2 (500-1000 µm): Tissue Volume Fraction in Percent.
Sub-Study 2 | New Bone | Lamellar Bone |
---|---|---|
Surface | Mean (SD) | Mean (SD) |
GB | 35.4 (6.7) | 11.2 (5.8) |
GB+AE+PS | 39.6 (6.1) | 9.3 (3.8) |
P-values | 0.502 | 0.473 |
Zone 1 | Mean (SD) | Mean (SD) |
GB | 18.3 (2.1) | 16.4 (6.4) |
GB+AE+PS | 17.5 (1.9) | 13.8 (4.1) |
P-values | 0.796 | 0.281 |
Zone 2 | Mean (SD) | Mean (SD) |
GB | 12.7 (4.4) | 18.4 (6.2) |
GB+AE+PS | 14.7 (6.5) | 16.7 (3.6) |
P-values | 0.468 | 0.530 |
There were no statistically significant differences between Grit Blasted and Grit Blasted+Acid Etched (sub-study 1), or Grit Blasted and Grit Blasted+Acid Etched+Plasma Sterilized (sub-study 2) in regards to any of the tissues compared in any of the zones measured.
All implants were well osseointegrated, with almost 50% of the implant surface in direct bone contact in sub-study 1 (upper site) and almost 40% implant to bone contact in sub-study 2 (lower site). Furthermore, there was no fibrous tissue in the bone-implant interface in sub-study 1, and only 5% in sub-study 2.
Biomechanical Results (Table 3)
Data of Mechanical Parameters: Strength, Energy, Stiffness
Strength (MPa) | Energy (kJ/m2) | Stiffness (MPa/mm) | |
---|---|---|---|
Sub-study 1 | Mean (SD) | Mean (SD) | Mean (SD) |
GB | 10.15 (1.61) | 1.05 (0.46) | 73.12 (21.14) |
GB+AE | 9.41 (0.90) | 1.18 (0.52) | 65.60 (13.82) |
P-values | 0.148 | 0.572 | 0.380 |
Sub-study 2 | Mean (SD) | Mean (SD) | Mean (SD) |
GB | 7.02 (3.21) | 0.61 (0.47) | 64.57 (24.41) |
GB+AE+PS | 6.95 (1.77) | 0.61 (0.31) | 63.67 (16.23) |
P-values | 0.944 | 0.969 | 0.925 |
By gross observation, all implants were well anchored in the bone. The mechanical testing confirmed this observation.
The implants in sub study 1, which were placed more proximally in the tibia were significantly better anchored in all three mechanical parameters (strength, energy and stiffness) than the implants in sub study 2 at the more distal tibial location. No statistically significant mechanical differences were found within the two individual sub studies.
DISCUSSION
The purpose of this study was to improve implant osseointegration and thereby long-term implant survival by two different approaches. One approach was applying micro-textural changes on gritblasted implant surfaces by acid etching. The other approach was the removal of surface-adherent pro-inflammatory agents by plasma sterilization.
We showed that neither etching alone, nor etching combined with plasma sterilization improved the osseointegration and the fixation of the grit blasted titanium implants in a press-fit canine implant model.
The canine implant model was chosen because canine cancellous bone closely resembles human cancellous bone [25]. The paired design of the two sub-studies eliminates the influence of interindividual differences between the test animals. All implants were placed extra-articularly and not exposed to direct loading, which limits this model in its representation of a clinical joint replacement. The model is, however, well established and has in numerous publications shown a good ability to detect subtle differences in mechanical fixation and osseointegration caused by surface modifications [26-28]. Grit-blasted implants were chosen because they represent a well-recognized, clinically applied surface for uncemented joint replacement components [29, 30].
Previous studies have shown positive effects of acid etching of titanium implants, using a similar canine implant model [26]. However, both the baseline treatment of the implants and the etching technology were different. Daugaard et al. used a dual etching technique on a chemically milled surface, whereas we used single etching on a commercial available grit blasted surface. Better performing control coatings together with a slightly increased under-drilling (0.1 mm – 0.5 mm) in our setting may explain the increased bone-to-implant contact in our study (more than 50% in both groups in sub-study 1) compared to Daugaard et al. (control: 18.4%, etched: 36.2%). This excellent degree of osseointegration may have been difficult to further improve.
As previously reported, we also observed that etching reduced the amount of grit blasting debris on the implant surface [31]. We did not see any short-term benefits of this in terms of osseointegration and early implant fixation.
Although clearing the implant surface of endotoxin theoretically would improve osseointegration, we found no positive effect of plasma sterilizing the etched implants. This may very well reflect the fact that there is a dose dependent response to endotoxin. Endotoxin tends to accumulate on polyethylene, and the negative effect of polyethylene wear particles can partly be ascribed to surface-adherent endotoxin [32].
Furthermore, after insertion of an endotoxin free implant, endogenous endotoxins will adhere to its surface. If an implant contaminated with exogenous endotoxin is inserted, some of the endotoxin will be cleared from the implant. This potentially establishes a comparable steady state concentration of endotoxin on the surface of both intervention and control implants [33].
Even though acid etching did not improve the initial osseointegration in this study, the reduction of alumina particles on the implant surface may prove beneficial long-term by reducing the amount of particulate metal in the periprosthetic tissue [34]. In clinical practice, only a small portion (10% to 20%) of the un-cemented press-fit implant surface is in bone contact [35]. Therefore improvement of the remaining 80% - 90% should be investigated in a model where ‘over-drilling’ introduces a small gap between the bone and the implant surface. Analysis of the bone/implant interface directly after implant insertion could be interesting to perform in future studies. This would provide a base line level of bone contact before the four weeks observation time. Furthermore, to test the effect of the bio burden, it would be relevant to perform a dose-response study.
CONCLUSION
Neither acid etching nor plasma sterilization of the grit blasted implants enhanced osseointegration or mechanical fixation in this press-fit canine implant model in a statistically significant manner. Two theoretically attractive ways of improving implants performance failed to prove efficiency in this press-fit canine implant model. All implants performed extremely well both in regards of osseointegration and mechanical fixation presenting a likely explanation for the lack of any proven benefits by acid etching and plasma sterilization.
ACKNOWLEDGEMENT
The authors would like to thank Depuy Orthopaedics Inc. for financial and material support. The authors would also like to thank laboratory technicians Jane Pauli and Annette Milton for excellent laboratory work.
CONFLICT OF INTEREST
The author(s) confirm that this article content has no conflicts of interest.