All published articles of this journal are available on ScienceDirect.
Comparative Effectiveness of Adjustable Lordotic Expandable versus Static Lateral Lumbar Interbody Fusion Devices: One Year Clinical and Radiographic Outcomes
Abstract
Aims:
This study aims to understand the clinical and radiographic outcomes between patients treated with static and expandable interbody spacers with adjustable lordosis for minimally invasive (MIS) lateral lumbar interbody fusion (LLIF).
Background:
The use of large interbody spacers in MIS LLIF offers favorable clinical and radiographic results. Static interbody spacers may cause iatrogenic endplate damage and implant subsidence due to forceful impaction and excessive trialing. Expandable interbody spacers with adjustable lordosis offer in situ expansion that may optimize endplate contact and maximize and maintain sagittal alignment correction until fusion occurs.
Objective:
The objective of this study is to compare the clinical and radiographic outcomes between patients treated with static and expandable interbody spacers with adjustable lordosis for MIS LLIF.
Methods:
This is a multi-surgeon, retrospective, Institutional Review Board-exempt chart review of consecutive patients who underwent MIS LLIF at 1-2 contiguous level(s) using either a polyetheretherketone (PEEK) static (32 patients) or a titanium expandable spacer with adjustable lordosis (57 patients). The mean differences of radiographic and clinical functional outcomes were collected and compared from preoperative up to 12-month postoperative follow-up. Statistical results were significant if P<0.05.
Results:
The mean improvement of VAS back pain scores from preoperative to 6 and 12 months was significantly higher in the expandable group compared to the static group (P<0.05). Mean improvement of Oswestry Disability Index (ODI) scores from preoperative to 3, 6, and 12 months were significantly higher in the expandable group compared to the static group (P<0.001). The expandable group had a significantly greater mean improvement in segmental lordosis from preoperative to 6 weeks, 3, 6, and 12 months (P<0.001). For disc height, the mean improvement from preoperative to 6 weeks and 3 months was more significant in the expandable group compared to the static group (P<0.05). In the expandable group, the mean improvement from preoperative to 6 weeks, 3, and 6 months was significantly greater compared to the static group for neuroforaminal height (P<0.001). Subsidence was 0% in the expandable group and 32.4% (12/37) in the static group.
Conclusion:
This study showed significant positive clinical and radiographic outcomes for patients who underwent MIS LLIF using titanium expandable interbody spacers with adjustable lordosis based on significant changes in VAS back pain scores, ODI scores, and radiographic parameters at 12-month follow-up. There was a 0% subsidence rate in the expandable group, compared to a 32% subsidence rate in the static group.
1. INTRODUCTION
McAfee et al. [1] and Bagby [2] popularized the interbody device for lumbar arthrodesis. There are a variety of surgical techniques and approaches including anterior lumbar interbody fusion, posterior lumbar interbody fusion, and transforaminal lumbar interbody fusion. Lateral lumbar interbody fusion (LLIF) is a popular retroperitoneal transpsoas approach that may help minimize the risk of complications associated with anterior and posterior lumbar interbody fusion techniques [3, 4].
Static interbody spacers may cause iatrogenic endplate damage and implant subsidence due to forceful impaction and excessive trialing [5-9]. Expandable interbody spacers with adjustable lordosis offer in situ expansion that may better restore disc height and segmental lordosis. The importance of restoring disc height and segmental lordosis in spinal arthrodesis has been well-established [10]. Restoration of lordosis has been correlated with improved functional outcomes [11-13]. However, there are only a small number of studies that compare functional outcomes of expandable to static interbody spacers [14-22]. This study compares the clinical and radiographic outcomes between patients treated with polyetheretherketone (PEEK) static and titanium expandable interbody spacers with adjustable lordosis for LLIF.
2. MATERIALS AND METHODS
This is a multi-site, multi-surgeon retrospective study from a prospectively collected cohort of patients who were diagnosed with symptomatic degenerative disc disease with or without grade 1 spondylolisthesis in 1 or 2 contiguous levels and underwent LLIF using a PEEK static interbody spacer with supplemental fixation, or minimally invasive (MIS) LLIF with a novel titanium expandable interbody spacer with adjustable lordosis (RISE-AL®; Globus Medical, Inc.) (Figs. 1 and 2) with supplemental fixation. Patient demographics, visual analog scale (VAS) back pain scores, Oswestry Disability Index (ODI) scores and radiographic parameters were collected at 6 weeks, 3, 6, and 12 months postoperatively. Raw radiographic measurements were collected, reported, and analyzed. The difference between baseline and each time point per patient was calculated and reported as ‘the mean difference’. This was calculated to help minimize the effect of the difference between the preoperative radiographic measurements between groups.
2.1. Surgical Technique
After induction of general anesthesia, patients were placed in the lateral decubitus position and secured to the operating table with adhesive medical tape. Under fluoroscopic guidance, an oblique incision was made at the operative disc segment. Blunt dissection was performed under direct visualization through the retroperitoneal space. Retroperitoneal fat was mobilized anteriorly, exposing the underlying psoas muscle. The psoas muscle was palpated, and blunt dissection was performed down to the operative intervertebral disc level. After confirmation of the appropriate level via fluoroscopy, a minimally invasive retractor was docked, dilated at the segment, and secured to the table-mounted arm. An annulotomy was then performed, followed by a discectomy. Under fluoroscopic imaging, the endplates were prepared.
2.2. Expandable Group
For the expandable group, an expandable trial was used to allow for the gradual distraction of the disc space. An expandable interbody spacer of appropriate size was selected, packed with autograft, and implanted laterally across the disc space. The spacer was then expanded to the desired height and backfilled with autograft Fig. (3).
The expandable interbody spacer used in this study is manufactured from titanium alloy. The device is inserted at a contracted height and expanded in situ once correctly positioned within the intervertebral space, offering continuous expansion and adjustable lordosis for optimal endplate-to-endplate contact.
2.3. Static Group
For the static group, the smallest static trial was inserted into the disc space, moving to larger trials as needed, allowing for the distraction of the disc space. A static interbody spacer of appropriate size was selected, packed with autograft, and implanted laterally across the disc space.
The static interbody spacer used in this study is manufactured from a PEEK radiolucent polymer with titanium alloy or tantalum markers.
2.4. Supplemental Fixation
In both groups, pedicle screws and rods were used for supplemental fixation. Locking caps were set once the rods were in their proper position. Intraoperative fluoroscopy images were taken to verify the screw and rod position. Surgical incisions were cleaned and closed in the standard fashion.
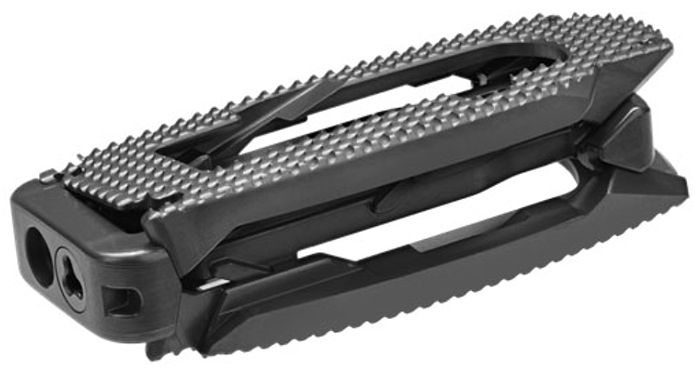
2.5. Quantitative Measurements
Radiographic lumbosacral parameters were measured on upright lateral radiographs using imaging software (Surgimap®; Nemaris, New York, NY, USA) Fig. (4). Measurements included disc height, neuroforaminal height, and segmental lordosis. Disc height was defined as the distance between the inferior and superior endplates at the middle portion of the vertebral body. Neuroforaminal height refers to the interpedicular height or the rostral and caudal boundaries of the foramen. Segmental lordosis was measured as the Cobb angle of the superior endplate of the level below the LLIF and the inferior endplate of the level above the LLIF.
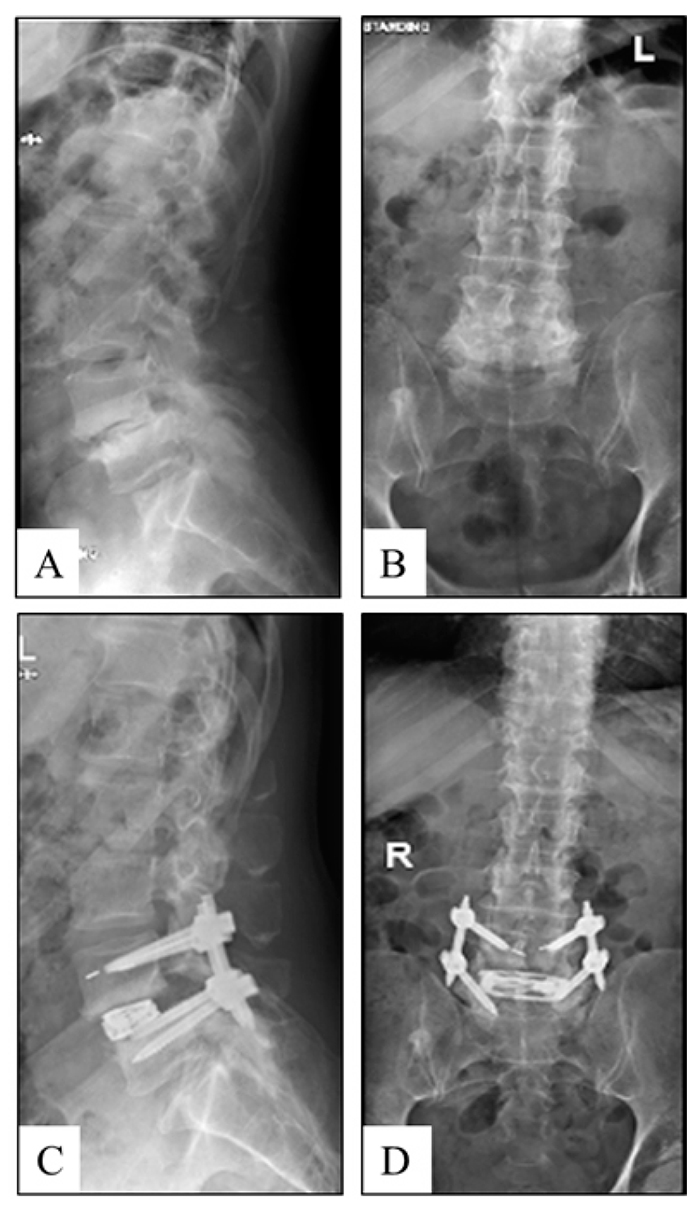
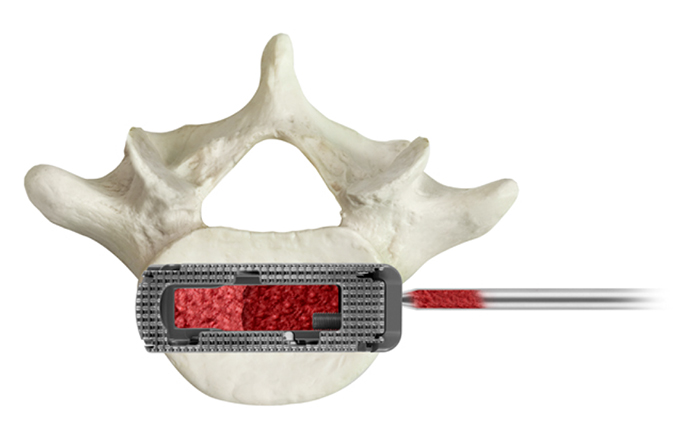
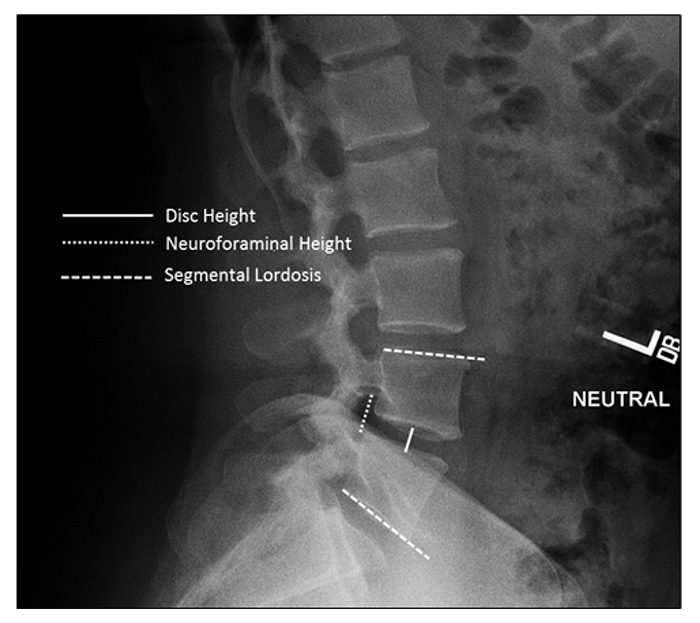
2.6. Statistical Analysis
The statistical analysis was performed using IBM® SPSS® Version 25 (IBM® Corp.; Armonk, NY, USA). Descriptive statistics are presented as frequencies and percentages. Clinical and radiographic measurements are presented as means and standard deviations. Statistical significance was shown at P<0.05.
3. RESULTS
3.1. Patient Demographics
The average age of the static group was significantly higher compared to the expandable group (P<0.001).
A total of 57 consecutive patients underwent MIS LLIF from August 2016 to November 2017 and were implanted with a titanium expandable interbody spacer with adjustable lordosis. The patients were 49.1% (28/57) female and 50.9% (29/57) male, with an average age of 58.0±12.3 years (range: 21-79 years).
A total of 32 consecutive patients underwent LLIF from May 2014 to February 2016 and were implanted with a PEEK static interbody spacer. The patients were 68.8% (22/32) female and 31.2% (10/32) male, with an average age of 66.3±8.9 years (range: 45-81 years) (Table 1).
3.2. Surgical Data
Of the 57 patients implanted with the titanium expandable interbody spacer with adjustable lordosis, 77.2% (44/57) underwent one-level and 22.8% (13/57) underwent two-level MIS LLIF, for a total of 70 spinal levels treated. Of the 70 levels, 45.7% (32/70) were performed at L4-L5 and 32.9% (23/70) were performed at L3-L4. The average estimated blood loss was 22.0±10.5cc for one-level fusions and 30.8±7.3cc for two-level fusions with no blood transfusions. For one-level fusions, the mean operative time was 53.4±11.0 min and 79.9±20.1 min for two-level fusions. The mean length of hospital stay was 3.3±2.0 days for one-level fusions and 4.5±2.8 days for two-level fusions.
Parameter | Expandable | Static |
---|---|---|
Number of Patients | 57 | 32 |
Gender | ||
Female, n (%) | 28 (49.1%) | 22 (68.8%) |
Male, n (%) | 29 (50.9%) | 10 (31.2%) |
Age, mean (SD, range) | 58 (12.3) (21-79) | 66.3 (8.9) (45-81) |
Of the 32 patients implanted with the PEEK interbody spacer, 71.9% (23/32) underwent one-level and 28.1% (9/32) underwent two-level LLIF for a total of 41 spinal levels treated. Of those 41 levels, 46.3% (19/41) were performed at L4-L5 and 36.6% (15/41) at L3-L4. The average estimated blood loss was 38.9±30.1 for one-level fusions and 86.7±155.9 for two-level fusions with no blood transfusions. For one-level fusions, the mean operative time was 65.0±39.6 min, and 84.1±32.2 min for two-level fusions. The mean length of hospital stay was 2.1±1.2 days for one-level fusions and 2.6±1.9 days for two-level fusions (Table 2).
Parameter | Expandable | Static |
---|---|---|
Type of Surgery, n (%) | ||
One-level | 44 (77.2%) | 23 (71.9%) |
Two-level | 13 (22.8%) | 9 (28.1%) |
Levels Instrumented, n (%) | ||
L1-L2 | 4 (5.7%) | 0 (0.0%) |
L2-L3 | 11 (15.7%) | 7 (17.1%) |
L3-L4 | 23 (32.9%) | 15 (36.6%) |
L4-L5 | 32 (45.7%) | 19 (46.3%) |
Mean Estimated Blood Loss, Mean (SD) | ||
One-level | 22.0 (10.5) | 38.9 (30.1) |
Two-level | 30.8 (7.3) | 86.7 (155.9) |
Mean Operative Time, Mean (SD) | ||
One-level | 53.4 (11.0) | 65.0 (39.6) |
Two-level | 79.9 (20.1) | 84.1 (32.2) |
Mean Length of Hospital Stay, Mean (SD) | ||
One-level | 3.3 (2.0) | 2.1 (1.2) |
Two-level | 4.5 (2.8) | 2.6 (1.9) |
3.3. Patient-Reported Outcomes (VAS and ODI)
The mean preoperative ODI score was significantly higher in the expandable group compared to the static group (P<0.001). There was no difference between the preoperative VAS scores (P=0.07).
The mean VAS back pain score significantly improved from baseline by 51.9% (4.2±1.0 points), 60.5% (4.9±1.2 points), 71.6% (5.8±1.2 points), and 80.2% (6.5±1.2 points), at 6 weeks, 3, 6, and 12 months, respectively, in the expandable group (P<0.001). Mean VAS back pain score significantly improved from baseline by 62.2% (4.7±2.3 points), 68.9% (5.1±2.3 points), 59.5% (4.3±2.9 points), and 70.3% (5.1±2.6 points) at 6 weeks, 3, 6, and 12 months, respectively, in the static group (P<0.001). For VAS back pain scores, the mean improvement from preoperative to 6 and 12 months was significantly greater in the expandable group compared to the static group by 29.7% and 24.1%, respectively (P<0.05) Fig. (5).
The mean ODI score significantly improved from baseline by 38.6% (29.9±13.1 points), 55.1% (42.6±12.2 points), 67.7% (52.4±13.5 points), and 78.9% (61.1±11.9 points) at 6 weeks, 3, 6, and 12 months, respectively, in the expandable group, and by 46.7% (24.3±24.4 points), 57.0% (28.8±20.6 points), 53.3% (26.8±22.6 points), and 59.2% (29.8±23.4 points), respectively, in the static group (P<0.001). For ODI scores, the mean improvement from preoperative to 3, 6, and 12 months was significantly greater in the expandable group by 38.7%, 64.7%, and 68.9%, respectively, compared to the static group (P<0.05) (Fig. 6 and Tables 3 and 4).
Parameter | Device | Baseline | 6 Weeks | 3 Months | 6 Months | 12 Months |
---|---|---|---|---|---|---|
VAS Back Pain | Expandable | 8.1 (0.7) | 3.9 (1.0)* | 3.2 (1.0)* | 2.3 (1.1)* | 1.6 (1.2)* |
Static | 7.4 (1.8) | 2.8 (2.5)* | 2.3 (2.2)* | 3.0 (2.9)* | 2.2 (2.6)* | |
ODI | Expandable | 77.3 (6.9) | 47.5 (14.5)* | 34.7 (12.3)* | 25.0 (13.0)* | 16.3 (11.7)* |
Static | 50.5 (19.2) | 26.9 (17.0)* | 21.7 (15.2)* | 23.6 (18.0)* | 20.6 (19.7)* | |
Middle Disc Height (mm) | Expandable | 6.4 (2.5) | 12.3 (2.2)* | 11.7 (2.4)* | 11.4 (2.3)* | 11.0 (2.2)* |
Static | 8.8 (2.8) | 13.4 (2.4)* | 12.9 (2.7)* | 12.9 (2.6)* | 12.6 (2.9)* | |
Neuroforaminal Height (mm) | Expandable | 14.1 (4.1) | 20.0 (3.8)* | 19.5 (3.6)* | 18.5 (3.4)* | 17.8 (3.0)* |
Static | 20.2 (4.1) | 22.6 (4.1)* | 22.5 (3.8)* | 21.9 (4.1)* | 22.3 (3.8)* | |
Segmental Lordosis (°) | Expandable | 4.7 (2.9) | 9.5 (3.4)* | 9.1 (3.1)* | 8.8 (3.0)* | 8.5 (2.6)* |
Static | 14.7 (8.5) | 15.2 (8.1) | 15.0 (8.7) | 14.9 (8.3) | 14.4 (8.5) |
Parameter | Device | 6 Weeks | 3 Months | 6 Months | 12 Months |
---|---|---|---|---|---|
VAS Back Pain | Expandable | 4.2 (1.0) | 4.9 (1.2) | 5.8 (1.2)* | 6.5 (1.2)* |
Static | 4.7 (2.3) | 5.1 (2.3) | 4.3 (2.9) | 5.1 (2.6) | |
ODI | Expandable | 29.9 (13.1) | 42.6 (12.2)* | 52.4 (13.5)* | 61.1 (11.9)* |
Static | 24.3 (24.4) | 28.8 (20.6) | 26.8 (22.6) | 29.8 (23.4) | |
Middle Disc Height (mm) | Expandable | 5.9 (2.4)* | 5.3 (2.3)* | 5.0 (2.3) | 4.7 (2.4) |
Static | 4.6 (2.8) | 4.1 (3.1) | 4.2 (3.0) | 3.9 (3.2) | |
Neuroforaminal Height (mm) | Expandable | 5.9 (3.9)* | 5.4 (4.0)* | 4.4 (4.0)* | 3.7 (3.9) |
Static | 2.5 (3.5) | 2.3 (3.9) | 1.8 (3.3) | 2.2 (4.3) | |
Segmental Lordosis (°) | Expandable | 4.8 (3.3)* | 4.4 (3.1)* | 4.1 (3.1)* | 3.8 (2.9)* |
Static | 0.6 (5.1) | 0.3 (5.1) | 0.2 (5.6) | -0.3 (4.6) |
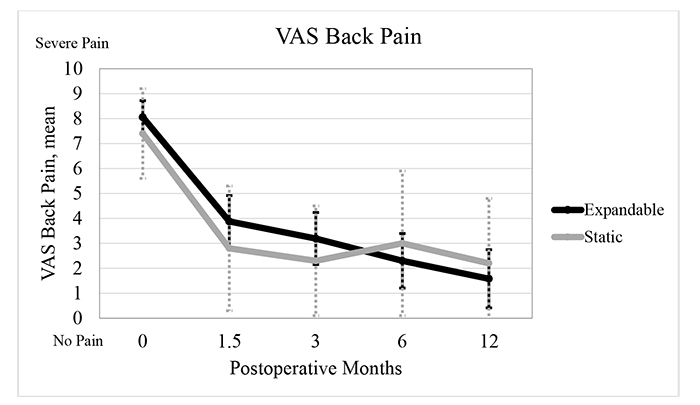
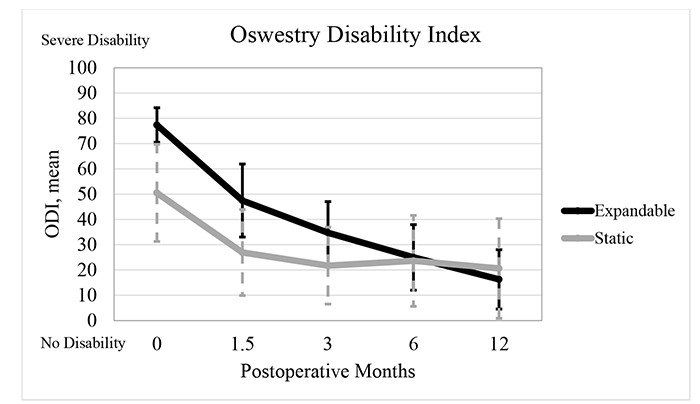
3.3.1. Radiographic Parameters
The mean preoperative segmental lordosis, disc height, and neuroforaminal height were significantly higher in the static group compared to the expandable group (P<0.001).
Segmental lordosis significantly improved from baseline in the expandable group by 102.1% (4.8±3.3°), 93.6% (4.4±3.1°), 87.2% (4.1±3.1°), and 80.9% (3.8±2.9°) at 6 weeks, 3, 6, and 12 months, respectively (P<0.001). Segmental lordosis improved from baseline in the static group by 3.4% (0.6±5.1°), 2.0% (0.3±5.1°), and 1.4% (0.2±5.6°) at 6 weeks, 3 months, and 6 months, respectively (P>0.05). Segmental lordosis decreased from baseline in the static group by 2.0% (-0.3±4.6) at 12 months (P>0.05). In the expandable group, the improvement in segmental lordosis was significantly greater by 155.6%, 174.5%, 181.4%, and 234.3%, at 6 weeks, 3, 6, and 12 months compared to the static group (P<0.001).
Disc height significantly improved from baseline in the expandable group by 92.2% (5.9±2.4 mm), 82.8% (5.3±2.3 mm), 78.1% (5.0±2.3 mm), and 71.9% (4.7±2.4 mm) at 6 weeks, 3, 6, and 12 months, respectively (P<0.001). Disc height significantly improved from baseline in the static group by 52.3% (4.6±2.8 mm), 46.6% (4.1±3.1 mm), 46.6% (4.2±3.0 mm), and 43.2% (3.9±3.2 mm) at 6 weeks, 3, 6, and 12 months, respectively (P<0.001). In the expandable group, the improvement in disc height was significantly greater by 24.8% and 25.5% at 6 weeks and 3 months, compared to the static group (P<0.05).
Neuroforaminal height significantly improved from baseline in the expandable group by 41.8% (5.9±3.9 mm), 38.3% (5.4±4.0 mm), 31.2% (4.4±4.0 mm), and 26.2% (3.7±3.9 mm) at 6 weeks, 3, 6, and 12 months, respectively (P<0.001). Neuroforaminal height significantly improved from baseline in the static group by 11.9% (2.5±3.5 mm), 11.4% (2.3±3.9 mm), 8.4% (1.8±3.3 mm), and 10.4% (2.2±4.3 mm) at 6 weeks, 3, 6, and 12 months, respectively (P<0.05). In the expandable group, the improvement in neuroforaminal height was significantly greater by 81.0%, 80.5%, and 83.9% at 6 weeks, 3 months, and 6 months compared to the static group (P<0.001) Tables 3 and 4.
Between groups, there was a significant difference between preoperative segmental lordosis, disc height, and neuroforaminal height (P<0.001).
4. DISCUSSION
Radiographic and clinical outcomes are essential to demonstrate the safety and effectiveness of expandable interbody spacers with adjustable lordosis in LLIF compared to static interbody spacers. At 12-month follow-up, radiographic outcomes of this study showed that MIS LLIF using titanium expandable interbody spacers with adjustable lordosis significantly corrected disc height and segmental lordosis by 71.9% and 80.9%, respectively, compared to a 43.2% increase in disc height and a 2.0% decrease in segmental lordosis observed with PEEK static interbody spacers.
To the best of the authors’ knowledge, this is the first study to describe the clinical and radiographic outcomes of the use of a titanium expandable interbody spacer with adjustable lordosis in MIS LLIF; therefore, comparison to the literature is challenging. In a prospective observational study, Marchi et al. [7] reported on the radiographic outcomes of patients who underwent stand-alone MIS LLIF for the treatment of low-grade degenerative spondylolisthesis with static PEEK interbody spacers. By 12-month follow-up, disc height increased by 61% and segmental lordosis increased by 62.9%, which is less than the reported radiographic outcomes of the current study for expandable interbody spacers with adjustable lordosis. Marchi et al. [7] reported a subsidence rate of 17.3%, where the expandable interbody spacer with adjustable lordosis in the current study has a subsidence rate of 0%.
In a case series of 140 consecutive patients (223 levels) treated with PEEK static interbody spacers and extreme lateral interbody fusion (NuVasive, Inc., San Diego, CA, USA) segmental lordosis improved by 28.0% at 12 months postoperative [23]. In a retrospective analysis by Kim et al. [15], segmental lordosis of “standard” static PEEK interbody devices (6° lordotic angle, 18mm width) improved by 11.0%, while segmental lordosis of the “wide” static PEEK interbody devices (12° lordotic angle, 22mm width) improved by 42.7%. In a similar analysis by Marchi et al. [8], segmental lordosis of the “standard” interbody devices improved by 1.1%, while the “wide” interbody devices group improved by 16.9% at 12-month follow-up. In the current study, segmental lordosis improved by 80.9% at 12-month follow-up for the titanium expandable interbody spacer with adjustable lordosis and which was 2-4 times higher than what was previously reported using PEEK interbody devices.
4.1. Study Limitations
Although this is a retrospective study with a small patient population, the results are consistent with findings from the literature. The groups compared in the study were reviewed at two different institutions, and the surgeons are from two different specialties (neurosurgery vs. orthopaedic surgery). Yet, both surgeons utilized similar surgical techniques. Radiographic measurements were completed by two different observers for each group. Preoperative radiographic measurements also differed between the two groups; however, comparing the mean differences helped minimize the effect of this heterogeneity. While this study only focused on a 12-month follow-up, a 24-month follow-up study is forthcoming.
CONCLUSION
This study showed significant positive clinical and radiographic outcomes for patients who underwent MIS LLIF using titanium expandable interbody spacers with adjustable lordosis, based on significant changes in VAS pain scores, ODI scores, and radiographic parameters at 12-month follow-up compared to the PEEK static group. There was no subsidence in the expandable group compared to a 32% subsidence rate in the static group.
LIST OF ABBREVIATIONS
LLIF | = Lateral Lumbar Interbody Fusion |
MIS | = Minimally Invasive |
ODI | = Oswestry Disability Index |
PEEK | = Polyetheretherketone |
VAS | = Visual Analog Scale |
ETHICAL APPROVAL AND CONSENT TO PARTICIPATE
As the research conducted for this manuscript was a retrospective study on patient data, compliance with the ethical standards of the responsible committee on human experimentation (institutional and national) and the Helsinki Declaration of 1975 was not required.
HUMAN AND ANIMAL RIGHTS
Not applicable.
CONSENT FOR PUBLICATION
Not applicable.
AVAILABILITY OF DATA AND MATERIALS
Not applicable.
FUNDING
Funding for this project was provided by the Musculoskeletal Education and Research Center (MERC), a Division of Globus Medical, Inc (GMI).
CONFLICT OF INTEREST
YML, ZH, and YIL receive research support from GMI. RF receives research support, royalties, and is a consultant for GMI. CL and JR have stock and are employees of GMI. JT has nothing to disclose. The titanium expandable interbody spacer with adjustable lordosis (RISE-AL®) is manufactured by GMI.
ACKNOWLEDGEMENTS
We would like to thank Mr. Howard Whitman and Ms. Leigh Ahrendtsen for their hard work on this research project.