All published articles of this journal are available on ScienceDirect.
Hybrid Biosynthetic Autograft Extender for Use in Posterior Lumbar Interbody Fusion: Safety and Clinical Effectiveness
Abstract
Autologous iliac crest bone graft is the preferred option for spinal fusion, but the morbidity associated with bone harvest and the need for graft augmentation in more demanding cases necessitates combining local bone with bone substitutes. The purpose of this study was to document the clinical effectiveness and safety of a novel hybrid biosynthetic scaffold material consisting of poly(D,L-lactide-co-glycolide) (PLGA, 75:25) combined by lyophilization with unmodified high molecular weight hyaluronic acid (10-12% wt:wt) as an extender for a broad range of spinal fusion procedures. We retrospectively evaluated all patients undergoing single- and multi-level posterior lumbar interbody fusion at an academic medical center over a 3-year period. A total of 108 patients underwent 109 procedures (245 individual vertebral levels). Patient-related outcomes included pain measured on a Visual Analog Scale. Radiographic outcomes were assessed at 6 weeks, 3-6 months, and 1 year postoperatively. Radiographic fusion or progression of fusion was documented in 221 of 236 index levels (93.6%) at a mean (±SD) time to fusion of 10.2+4.1 months. Single and multi-level fusions were not associated with significantly different success rates. Mean pain scores (+SD) for all patients improved from 6.8+2.5 at baseline to 3.6+2.9 at approximately 12 months. Improvements in VAS were greatest in patients undergoing one- or two-level fusion, with patients undergoing multi-level fusion demonstrating lesser but still statistically significant improvements. Overall, stable fusion was observed in 64.8% of vertebral levels; partial fusion was demonstrated in 28.8% of vertebral levels. Only 15 of 236 levels (6.4%) were non-fused at final follow-up
INTRODUCTION
With increasing understanding of the biological and biomechanical basis of bone fusion, the quest for better and more efficient bone fusion technologies in spinal surgery continues. While autologous iliac crest bone is recognized as the most successful grafting material due to its osteoconduc-tive, osteogenic and osteoinductive properties [1, 2], compli-cation rates of this invasive procedure remain high [3-5], with protracted postoperative pain at the donor site being the most commonly reported complication [6]. In an attempt to limit such complications by either reducing or eliminating iliac crest autograft harvest, demineralized bone matrix (DBM) and synthetic osteoconductive bone graft extenders (BGEs) are often used in spinal fusion procedures as an adjunct to patient-derived autograft [7-11]. Similarly, local bone may be used in lieu of iliac crest grafts, although current evidence suggests that while local bone is equally effective as iliac crest bone in single-level fusion [6, 12], it has been associated with significantly lower fusion rates when utilized in multi-level fusion due to volume limitations [1].
A number of bone graft substitutes are available, the most common of which are ceramic- or calcium-phosphate-based synthetic materials. While these products provide a suitable scaffolding upon which to build new bone, they are inherently unable to stimulate bone growth without the patient’s own bone or marrow to augment the process. Alternate options such as tricalcium phosphate, calcium sulfate and hydroxyapatite, while possessing osteoconduc-tive properties, are associated with equivocal results regarding fusion rates [13]. To address the shortcomings of the currently available technology, a fully-resorbable hybrid biosynthetic autograft extender for use in spinal fusion was developed (InQu®, ISTO Technologies, Inc., St. Louis, MO). This extender, which has been commercially available since 2008, contains no ceramic component and no animal-derived protein. Instead, it consists of poly(D,L-lactide-co-glycolide) (PLGA, 75:25) combined by lyophilization with unmodified high molecular weight hyaluronic acid (10-12% wt:wt) to create a hybrid interwoven scaffold. The PLGA component provides compressive resistance to maximize the product's bulking properties. The rate of polymer resorption (3-6 months) is consistent with the rate of bone remodeling at the site of implantation, and this scaffolding support has been shown to direct new bone growth via endochondral ossification [14, 15], in contrast to ceramic-based materials, which function through creeping substitution [16].
In order to evaluate the safety and clinical effectiveness of the new hybrid interwoven biosynthetic bone extender, we retrospectively analyzed a consecutive series of posterior lumbar interbody fusion (PLIF) procedures with supplemental instrumentation.
METHODS
All patients who underwent elective PLIF procedures at a single academic medical center between March 2008 and March 2011 utilizing the new hybrid interwoven biosynthetic bone extender were included in this analysis. All patients signed an institutional consent form that explained all treatment modalities offered to these patients. No Institutional Review Board (IRB) review was sought as all procedures were performed under our center’s standard of care and did not involve any additional research-oriented procedure or products.
One author (MKC) performed a retrospective chart review of eligible patients. The goal of the review and data collection was to document the rate of fusion progression at approximately 18 months of follow-up together with the rate of complications and changes in pain scores and other patient-reported outcomes.
Surgical Procedure
Surgery was performed using standard surgical techniques of decompression and fusion. In all cases, local bone was harvested during the decompression procedure using an irrigated high speed burr and mucous trap. The harvested bone was combined with concentrated bone marrow aspirate (cBMA) obtained from the posterior iliac crest. In the vast majority of cases (95/109, 87%), the local bone/cBMA mixture was first extended with a cancellous crunch allograft (5-10 cc) before addition of the hybrid interwoven biosynthetic bone extender (5-10 cc). DBM was additionally used in 55 cases (50%). The formulation of InQu (granules or paste mix) was selected for each individual patient based on the amount of bulk necessary to fill the bone void. Nearly all cases (95/109, 87%) used 10 cc of paste mix, while 10 cases (8.7%) utilized granules. The formulation used was not recorded in 4 cases.
In all patients, the bone grafting material was placed into the interbody space and the posterolateral gutters as per standard instrumentation procedures. Disc spaces were augmented with 15 cc of bone graft material prior to interbody placement. Interbody cages were used in all cases, with the disc space filled to its entirety. Constructs varied from 1 to 7 intervertebral levels with all cases supported by standard instrumentation (standard rods, screws and when necessary, crosslinks).
Outcomes
Patient Reported Outcomes – Pain severity were reported subjectively using a visual analog scale (VAS) ranging from 0 (no pain) to 10 (worst pain imaginable). The minimum improvement in VAS required to indicate a minimally clinically important difference (MCID) ranges from 1.3 [17] to 3.0 [18], depending on study and field. For the purposes of this analysis, we set our MCID at an improvement of 2.0 points on the 10-point VAS, which is consistent with similar studies from this field [19-22].
Radiographic Outcomes – Radiographs were obtained at baseline and post-procedure at 6 weeks, 3-6 months, and 12 months; computed tomography (CT) scans were collected 3-6 months and 12 months post-procedure. Independent board-certified neuroradiologists reviewed both static and dynamic radiographs and CT scans to determine fusion status. In all cases, fusion status was defined by the presence of continuous bridging bone between the endplates. Delayed or non-union was characterized by the presence of visualized intervertebral clefts or a vacuum-phenomenon [23]. Fusion was rated as either 0 (non-fusion), 1 (incomplete or progression of fusion) or 2 (stable/solid fusion), with procedures rated either “1” or “2” on this scale deemed to have been successful.
Outcomes for safety were evaluated by recording the nature and frequency of all adverse events that required medical attention or reoperation.
Statistical Analysis
Alpha was set a priori at 0.05 for all statistical comparisons. Means were compared using Student’s t-tests assuming unequal variances and/or single-factor ANOVA. All mean values are presented as mean (±SD). VAS and VAS responder rates are presented as mean value (±SD).
RESULTS
One hundred nine (109) procedures were performed on 108 patients involving 245 individual vertebral levels. Patient background characteristics and pre-surgical diagnoses are summarized in Table 1. The mean age was 59.4 (±12.4 yrs, range 28-85 yrs); 56% of patients were female. Only 39 patients (36%) reported working prior to the surgery. Mean body mass index (BMI) was 30.2 (±5.7). Mean clinical follow-up was 19.2 (±11.7) months, whilemean radiological follow-up was 17.7 (±10.4) months. Three patients (2.8%), accounting for a total of 9 fusion levels, did not return for full follow-up. Of the initial 245 levels fused, complete data are available for 236 levels.
Patient characteristics and diagnosis.
All Patients (n=108) | |
---|---|
Mean age at time of first surgery | 59.4 (12.4) (range: 28- 85) |
Gender | |
Male | 47 (44%) |
Female | 61 (56%) |
Mean BMI at time of surgery | 30.2 (5.7) |
Diagnosis | |
Degenerative spinal stenosis | 58 (53%) |
Degenerative spondylolisthesis | 60 (55%) |
Non-specific low back pain | 18 (17%) |
Degenerative scoliosis | 6 (6%) |
Instability | 3 (3%) |
Radiculopathy | 4 (4%) |
Degenerative disc disease | 3 (3%) |
Post laminectomy syndrome | 1 (1%) |
Details of spine fusion procedure.
All Procedures (N=109) |
|
---|---|
Fusion type | |
Posterior lumbar interbody fusion (PLIF) | 109 (100%) |
Number of operated intervertebral levels | |
1 | 32 (29.4%) |
2 | 37 (33.9%) |
3 | 30 (27.5%) |
4 | 6 (5.5%) |
5 | 1 (0.9%) |
6 | 1 (0.9%) |
7 | 2 (1.8%) |
Source of bone | |
Local autograft (bone dust) | 109 (100%) |
Autologous bone marrow cBMA | 109 (100%) |
Extenders | |
Cancellous allograft extender | 95 (87%) |
Demineralized bone matrix | 55 (50%) |
Other (injectable protein polymer formulation *) | 1 (1%) |
InQu formulation used | |
Paste | 95 (87%) |
Granules | 10 (9%) |
Not recorded | 4 (4%) |
* NuCoreTM Injectable Disc Nucleus, Protein Polymer Technologies, Inc., San Diego, CA.
Abbreviations: cBMA: concentrated bone marrow aspirate.
Demographic characteristics sub-grouped based on number of index levels fused.
Single-Level (n=32 pts, 32 levels) |
Two-Level (n=37 pts, 74 levels) |
Multi-Level (n=40 pts, 139 levels) |
p-Value (ANOVA) |
|
---|---|---|---|---|
Age, mean (SD) | 58.1 (14.4) | 56.0 (11.8) | 63.7 (10.1) | 0.066 |
BMI, mean (SD) | 28.8 (5.6) | 29.5 (4.6) | 39.1 (6.4) | 0.041 |
Blood loss (mL), mean (SD) | 127 (47) | 230 (102) | 426 (245) | <0.00012 |
Length of stay (days), mean (SD) | 3.7 (1.7) | 4.2 (1.5) | 4.9 (2.6) | 0.051 |
1 Single level vs multi-level comparison: p=0.027. All other paired comparisons: p>0.05.
2 p<0.0001 for ANOVA and all paired t-tests.
Final follow-up observed fusion rates based on number of levels fused.
Full Follow-Up Procedures (n=105 Patients, 236 Levels) | |
---|---|
Time to last follow-up CT scan | |
Mean (SD), months | 17.7 (10.4) |
Median (Min, Max), months | 17.1 (1, 45.5) |
Time to stable or progression of fusion | |
Mean (SD), months | 10.2 (4.1) |
Median (Min, Max), months | 10 (1, 24) |
All cases, n (%) | |
Stable or progression | 221/236 (93.6) |
Stable/good | 153/236 (64.8) |
Incomplete/progression | 68/236 (28.8) |
Non-fusion | 15/236 (6.4) |
Stable or progression rate by number of levels involved, n (%) | |
1-level cases (n=32), overall | 30/32 (93.8) |
Partial/incomplete fusion | 8/32 (25) |
Complete fusion | 22/32 (68.8) |
2-level cases (n=36), overall | 62/72 (86.1) |
Partial/incomplete fusion | 12/72 (16.1) |
Complete fusion | 50/72 (69.4) |
3-level cases (n=30), overall | 84/87 (96.6) |
Partial/incomplete fusion | 42/87 (48.3) |
Complete fusion | 42/87 (48.3) |
4-level cases (n=5), overall | 20/20 (100) |
Partial/incomplete fusion | 0/20 (0) |
Complete fusion | 20/20 (100) |
5-level cases (n=1), overall | 5/5 (100) |
Partial/incomplete fusion | 0/5 (0) |
Complete fusion | 5/5 (100) |
6-level cases (n=1), overall | 6/6 (100) |
Partial/incomplete fusion | 6/6 (100) |
Complete fusion | 0/6 (0) |
7-level cases (n=2), overall | 14/14 (100) |
Partial/incomplete fusion | 0/7 (0) |
Complete fusion | 14/14 (100) |
Fusion success rates, based on fused level, for cases utilizing DBM and those not utilizing DBM.
DBM (122 Levels) | Non-DBM (114 Levels) | |||
---|---|---|---|---|
Overall Success1 Rate, n (%) | Detailed Fusion Rates, n (%) | Overall Success Rate, n (%) | Detailed Fusion Rates, n (%) | |
Single-level fusion | 14/14 (100) | No fusion: 0/14 (0) Partial: 3/14 (21) Complete: 11/14 (79) |
16/18 (87) | No fusion: 2/18 (13) Partial: 5/18 (27.8) Complete: 11/18 (61) |
Two-level fusion | 38/44 (86.4) | No fusion: 6/44 (13.6) Partial: 8/44 (18.2) Complete: 30/44 (68.2) |
24/28 (85.7) | No fusion: 4/28 (14.3) Partial: 4/28 (14.3) Complete: 20/28 (71.4) |
Multi-level fusion | 64/64 (100) | No fusion: 0/64 (0) Partial: 27/64 (42.2) Complete: 37/64 (57.8) |
65/68 (95.6) | No fusion: 3/68 (4.4) Partial: 21/68 (30.9) Complete: 44/68 (64.7) |
1 Overall success defined as patient achieving partial or complete fusion at final follow-up.
DBM: demineralized bone matrix.
Pain severity responder rates.
Pre-op VAS, Mean (SD) |
Post-op VAS, Mean (SD)1 |
12-Month Post-Op VAS, Mean (SD)1 |
Patients Achieving MCID Immediately Post-Op, n (%) |
Patients Achieving MCID 12-Months Post-Op, n (%) |
|
---|---|---|---|---|---|
All patients | 6.8 (2.5) | 3.1 (3.0) | 3.6 (2.9) | 63/90 (70.0) | 36/54 (66.7) |
Single-level | 6.6 (2.8) | 2.8 (3.1) | 2.7 (3.1) | 18/26 (69.2) | 10/15 (66.7) |
Two-level | 6.1 (2.6) | 2.6 (3.0) | 3.2 (2.5) | 18/26 (69.2) | 12/18 (66.7) |
Multi-level | 7.6 (1.8) | 3.8 (2.9) | 4.8 (3.0) | 23/33 (69.7) | 12/18 (66.7) |
1 p<0.001 for all pre-op vs post-op comparisons.
Pre-op: pre-operative.
Post-op: post-operative.
VAS: visual analog scale.
MCID: minimally clinically important difference.
Post-procedural re-operations.
Type of Events | Number of Events |
---|---|
Hardware removal/replacement | 12 |
Extension of construct to adjacent levels | 9 |
Laminectomy, foraminotomy and/or decompression | 3 |
Hematoma | 2 |
Adjacent level decompression | 2 |
Failure | 1 |
Infection | 1 |
Augmented fusion | 1 |
PLIF procedures at all 245 levels were instrumented. A detailed summary of the distribution of index levels is provided in Table 2; procedural details are summarized in Table 3. Spinal fusion was performed at a single level in 32 patients (29.4%), at two levels in 37 patients (33.9%) and at three or more levels in 40 patients (36.7%).
Clinical Outcomes, Time to Fusion and Fusion Rate
Data at final follow-up were available for 236 of 245 individual levels in 105 patients. A total of 221 of 236 vertebral levels (93.6%) fulfilled the success criteria for fusion at final follow-up by demonstrating partial or comp-lete fusion (see Table 4). Partial fusion was demonstrated at 68 of 236 fusion levels (28.8%) at final follow-up while 153 of 236 levels (64.8%) demonstrated complete fusion. Only 15 of 236 levels (6.4%) showed no evidence of fusion at final follow-up. The mean time to fusion for the entire group was 10.2 months (±4.1). An example of solid fusion is demonstrated in Fig. (1).
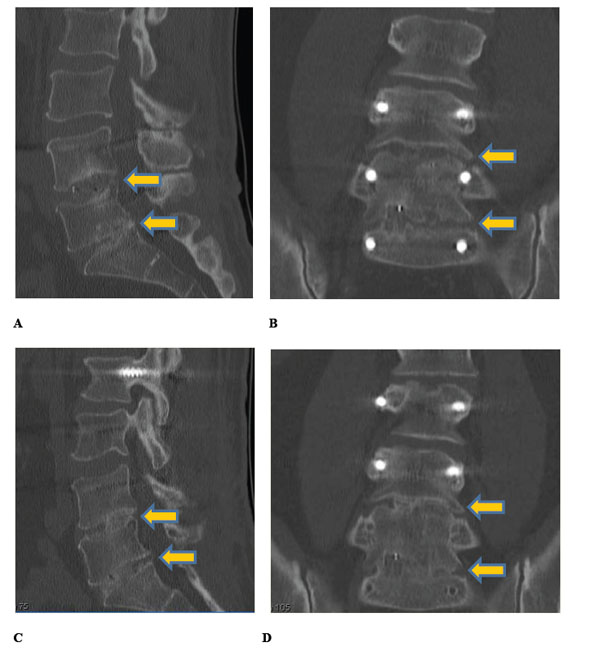
Sagittal (A, C) and coronal (B, D) CT images at 9 months (A, B) and 16 months (C, D) post-op in a 54-year-old male who underwent 2-level posterior lumbar interbody fusion at L4-S1 (yellow arrows). The patient complained of low back pain and left buttock pain after failed previous fusion at L5-S1. Nine-month imaging demonstrates progression to fusion (A, B), with complete fusion realized by 16-month imaging at the time fusion was extended to include L2-L4 (C, D). Low back pain and radiculopathy resolved following re-operation.
Single-Level Fusion
Spinal fusion was performed at a single level in 32 patients (29.4%). The majority of these procedures (21) were performed at the L4/L5 level, with 4 procedures performed at the L3/L4 level and 7 performed at the L5/S1 level. The mean age of this subgroup was 58.1 yrs (±14.4) with a mean BMI of 28.8 (±5.6). Mean blood loss in this subgroup was 127 mL (±47) while mean LOS was 3.7 days (±1.7).
Spinal fusion was rated as successful (complete or partial fusion) in a total of 30 (93.8%) single-level patients. Non-fusion was observed in only 2 (6.3%) patients. Of those patients deemed successfully fused, progression toward or partial fusion was observed in 8 (25%), while 22 patients (68.8%) demonstrated stable or complete fusion. The mean time to fusion in this group was 10.7 months (± 3.9).
The use of DBM was associated with a higher overall success rate as compared to cases where DBM was not utilized. In cases where DBM was utilized (n=14), 100% of patients were found to fulfil the success criteria for fusion, 21% (3/14) of which demonstrated partial fusion and 79% (11/14) demonstrated complete fusion. In contrast, in cases where DBM was not utilized (n=18), 87% of patients demonstrated successful fusion, with 28% (5/18) showing partial fusion and 61% (11/18) showing complete fusion. No patients in the group using DBM showed non-fusion at the final follow-up whereas 2 (13%) of those who did not utilize DBM were considered non-fused (see Table 5).
Two-Level Fusion
A total of 37 patients underwent spinal fusion at two levels. The majority of these procedures (26) were performed between L4 and S1. Ten procedures were performed between L3 and L5, with one procedure occurring between L2 and L4. The mean age of this subgroup was 56.0 yrs (± 11.8). Mean blood loss was 230 mL (±102); mean LOS was 4.2 days (±1.5) and mean BMI was 29.5 (±4.6).
Successful fusion was observed in 31 of 36 (86.1%) of patients (one patient in this group was lost to follow-up). Six patients (16.7%) were deemed to have progression toward or partial fusion while 25 (69.4%) were classified as stable or complete fusion. Non-fusion was observed in 5 (13.9%) patients. The mean time to fusion was 9.5 months (±3.9).
The use of DBM in two-level fusions was not associated with better success rates. Patients where DBM was utilized showed an 86% overall success rate (4/22 (18%) partial fusion and 15/22 (68%) complete fusion), while cases not using DBM showed an 85% overall success rate (2/14 (14%) partial fusion and 10/14 (71%) complete fusion).
Multi-Level Fusion
Fusion at greater than 2 levels occurred in 40 patients (see Table 2). The majority of patients were fused at 3 levels (30/40, 75%), with 10 additional patients undergoing fusion at greater than 3 levels. The mean age of this group was 63.7 yrs (±10.1). Mean blood loss was 426 mL (±245); mean LOS was 4.9 days (±2.6); and mean BMI was 31.9 (±6.4).
Two patients in this group were lost to follow-up and had no final fusion status. In the remaining 38 patients, fusion was deemed successful in 37 (97.4%). Only 1 patient (2.6%) was categorized as not fused. Of the successful fusion patients, 15 (39.5%) were observed to have progression toward or partial fusion, while 22 (57.9%) were categorized as stable or complete fusion. The mean time to fusion was 10.4 months (±4.4).
Fusion at three levels was performed in 29 patients, of whom 96.6% (28) had partial or complete fusion. Four-level fusion occurred in 6 patients, 1 of whom was lost to follow-up. Of the remaining 5 patients, all demonstrated partial or complete fusion at the final follow-up. Five- and six-level fusion was performed in 1 patient each while 2 patients underwent seven-level fusion. At the final follow-up, the patients undergoing 5- and 7-level fusion demonstrated complete fusion while the patient who underwent 6-level fusion demonstrated partial fusion.
The use of DBM had no effect on fusion rate in multi-level fusion cases. The overall success rate for fusion in the nine cases where greater than two levels were fused was 100% in all but one case, which demonstrated a 94% success rate with no DBM at 3-levels.
Patient-Reported Pain
Mean pain scores (±SD), as measured by VAS, improved significantly both immediately post-surgery and at 12-month follow-up. Overall, there was a statistically significant decrease in VAS both immediately post-operatively (6.8±2.5 vs 3.1±2.9, p<0.001) and 12-months post-operatively (6.8±2.5 vs 3.6±2.9, p<0.001), when compared with baseline VAS. Immediately post-surgery, 70.0% of all patients had realized a MCID in VAS scores. This improvement was consistent across all patients, regardless of the number of operative levels. Detailed patient responder rates are summarized in Table 6.
Adverse Events and Re-Interventions
No complications arising from the specific use of the hybrid interwoven biosynthetic bone extender were recorded in any of the 109 cases. Furthermore, no seromas and no heterotopic bone formation were observed. Re-operations performed during the follow-up period are summarized in Table 7. Procedural complications requiring medical attention and/or return to surgery included one patient seen for superficial infection at 3 months who was treated and released. Two additional patients developed hematoma and were treated immediately without neurologic sequelae. No cases of subsidence were reported. In addition, over the course of the follow-up period, 12 re-interventions were performed for hardware removal or replacement, and 9 patients required an extension of their surgical construct due to progressive degeneration of the spine.
Of the 39 patients who reported to be working prior to surgery, 56% (22/39) successfully returned to work.
DISCUSSION
The objective of this retrospective review was to investigate the safety and clinical effectiveness of InQu, a novel biosynthetic osteoconductive scaffold used as a local autograft extender in PLIF with supplemental instrumentation. This retrospective case series with radiologic assessment of fusion comprised 109 cases in 108 subjects at 245 individual lumbar spine levels. Local bone harvested during the decompression procedure was combined with the hybrid interwoven biosynthetic bone extender and this mixture was hydrated with concentrated bone marrow harvested from the posterior iliac crest. Posterolateral fusion was then achieved utilizing the same grafting materials placed within the interbody (IB) device and into the disc space.
The new hybrid interwoven biosynthetic bone extender appears to safely support bone formation when applied to the axial skeleton. A total of 64.8% of the 245 individual vertebral levels demonstrated complete and stable fusion at final follow-up, with an additional 28.8% of vertebral levels demonstrating progression toward fusion. Thus, a total of 221 of 236 vertebral levels (93.6%) fulfilled the success criteria for fusion, while only 15 of 236 levels (6.4%) showed lack of fusion at final follow-up. The mean time to fusion was an acceptable 10.2 months. No safety concerns were raised with use of the product, including the lack of heterotopic ossification. There were no reported cases of implant failure, deep wound infection or seroma formation post-surgery. No loosening or failure of the hardware was noted.
While technically more difficult and typically associated with greater complication rates than anterior lumbar interbody fusion (ALIF) and transforaminal lumbar interbody fusion (TLIF) procedures [24-26], the PLIF procedure – first pioneered in the 1950s [27-29] – is associated with high fusion rates, which has resulted in its remaining a staple of lumbar fusion. The current case series demonstrated satisfactory fusion rates in patients necessitating mechanical stabilization of single, double and multiple levels without neurological complication. Previous reports have suggested that the use of local bone in cases of multiple level fusion is associated with poor fusion outcomes, necessitating the use of iliac bone grafts [1, 30]. The current study, however, demonstrates successful fusion in 37 of 38 patients undergoing fusion at 3 or more levels (representing 129 of 132 levels). This indicates that the use of local bone in multi-level fusion is feasible when combined with an extender such as InQu. These data complement previous studies that have shown similar levels of fusion success when utilizing local bone. Coutour et al. [31] utilized the HYDROSORB bio-absorbable implant packed with locally harvested autograft and segmental internal fixation in a group of 27 patients undergoing PLIF and showed comparable efficacy, with 95.5% (42 of 44) of vertebral levels demonstrating successful fusion. They noted, however, that the rate of fusion was inversely proportional to the number of levels being fused, whereas we observed a nearly equivalent rate of fusion in single-, double- and multi-level fusion cases.
The fusion rates demonstrated in this report are comparable to those observed with the use of other bone graft extenders and fusion performed without BGE. Fusion rates above 85% at 2 to 3 years post-procedure have been demonstrated for both local and iliac crest bone grafts [12, 32]. Synthetic BGEs have been associated with similar rates of fusion success, with one recent systematic review [7] reporting an 86.4% fusion rate when ceramic materials were utilized as the BGE, while another systematic review [13] found pooled fusion rates ranged from 75% with calcium sulphate to 98.2% with a combination of hydroxyapatite and tricalcium phosphate. The current study included supplemental posterolateral fusion for which greater quantities of bone graft are required. Despite the finite volume of autograft that could be harvested locally, concentrated bone marrow aspirate combined with cancellous allograft and biosynthetic extender appeared to provided adequate osteogenic and osteoconductive support of new bone growth.
In conclusion, the clinical experience of a single clinical site in a 109 case series of subjects requiring PLIF procedures using a fully-resorbable osteoconductive scaffold made of PLGA with integrated hyaluronic acid demonstrates that this biosynthetic bone graft extender provides good to excellent radiographic outcomes in approximately 94% of patients. Most importantly, this study demonstrates that the use of the InQu extender allows for the use of local bone and resulted in a 97.7% fusion rate in 38 patients undergoing multiple level (>3) fusion. Additional prospective studies evaluating its clinical success for one- and two-level transforaminal lumbar interbody fusion procedures will add to these clinical findings.
ABBREVIATIONS
CONFLICT OF INTEREST
Jeffrey M. Muir and Jon E. Block received financial support from Henry Ford Health System (Detroit, MI) to assist with the preparation of this manuscript.
ACKNOWLEDGEMENTS
Henry Ford Health System (Detroit, MI) received financial support from ISTO in form of a grant to review clinical outcomes.