All published articles of this journal are available on ScienceDirect.
A Smart Tool for Intraoperative Leg Length Targeting in Total Hip Arthroplasty: A Retrospective Cohort Study
Abstract
Background:
Leg length discrepancies following total hip arthroplasty (THA) may necessitate subsequent interventions, from heel lifts to revision surgery. Current intraoperative methods of determining leg length are either inaccurate or expensive and invasive.
Objective:
The objective of the current study was to investigate the ability of a novel, intraoperative smart tool (Intellijoint HIP®, Intellijoint Surgical®, Inc., Waterloo, ON) to provide accurate, real-time leg length measurements during THA.
Methods:
We retrospectively reviewed the medical records of 25 patients who underwent THA utilizing the Intellijoint HIP smart tool between February and August 2014. Intraoperative leg length data was compared with radiographic leg length calculations. Two observers blinded to the Intellijoint HIP findings independently assessed all post-procedure radiographs.
Results:
The mean difference between smart tool and radiographic measurements was 1.3 mm [CI: -0.1, 2.7]. 88% (22/25) of intraoperative measurements were within 5 mm of radiographic measurements; 100% (25/25) were within 10 mm. A Bland-Altman analysis showed excellent agreement, with 96% (24/25) of measurements within the statistical limit for acceptable agreement, and 84% (21/25) within the clinically acceptable range (± 5 mm). Removal of the first 13 procedures (surgeon training) decreased the mean difference between methods to 0.6 mm [-0.6, 1.9]. All post-training procedures were associated with a difference of <5 mm. There were no reported adverse events related to the use of the smart tool.
Conclusion:
The Intellijoint HIP smart tool is a safe and accurate tool for providing intraoperative measurements of leg length in real-time.
INTRODUCTION
Leg length discrepancies (LLDs) following total hip arthroplasty (THA) are reported in up to 30% of primary procedures [1, 2], varying from 3 to 70 mm [3]. Post-THA LLD is associated with sequelae such as gait alteration [4-7], low back pain [8], neurological deficits [9-11] and patient dissatisfaction [12]. Leg length inequalities often necessitate corrective procedures, ranging from the use of contralateral heel lifts to full revision surgery, and have been associated with an increased risk of medical malpractice litigation [13, 14]. The hospital costs related to revision surgery can be substantial, with per-procedure costs reaching $29,000 in the United States [15] and £22,000 in Europe [16].
Surgeons traditionally lack accurate intraoperative data regarding changes in leg length during HTA procedures. Leg length has historically been assessed intraoperatively via manual methods, such as mechanical pins, osteotomy or tissue tensioning, methods that are subject to inaccuracies due to flexion contractures and inconsistencies in patient position during the procedure [17, 18]. Errors of up to 8 mm have been noted intraoperatively when introducing only 5 degrees of flexion or abduction to the leg [19].
In an attempt to improve intraoperative accuracy, sophisticated navigation devices have been developed to more accurately track changes in leg length. These devices, modified from their initial applications in neurological surgery [20-22], improve accuracy but have several drawbacks. The cumbersome and time-consuming nature of navigation systems can significantly increase surgical time [23, 24] while also increasing the risk of infection [25]. The costs associated with navigation, including capital costs of up to $250,000 USD, plus software costs and service contracts [26-28], result in increased per-procedure costs [27, 29] that can be prohibitive [28]. These limitations have contributed to the low rate of use for navigation in THA procedures, currently estimated at only 1-3% [30, 31].
To address the shortcomings of the existing technology, a novel miniature navigation tool has been developed to provide real-time, intraoperative data regarding leg length to surgeons. The Intellijoint HIP® smart tool (Intellijoint Surgical®, Inc., Waterloo, ON) is an intraoperative guidance tool used to assist orthopaedic surgeons in assessing leg length and offset changes during total hip arthroplasty. With minimal invasiveness and without altering surgical techniques or workflow, the device enables faster decision-making (fewer trial reductions) and minimizes leg length discrepancies. The Intellijoint HIP smart tool has been shown in cadaveric studies (data not published) to match the accuracy of radiographic leg length measurement. The purpose of this study was to investigate the ability of the Intellijoint HIP smart tool to accurately measure leg length intraoperatively, when compared with radiographic measurement, and to document any complications and/or adverse events associated with the device.
METHODS
Study Design
This study was a retrospective review of patients who underwent total hip arthroplasty, performed by two surgeons (PG, MS), utilizing the Intellijoint HIP smart tool.
Patient Eligibility
Patients eligible for inclusion in this study underwent a primary total hip arthroplasty procedure between February 2014 and August 2014 at a single community hospital. Specific inclusion criteria included: primary total hip arthroplasty; use of the Intellijoint HIP smart tool; ability to obtain pre and post-operative standing anterior-posterior (AP) radiographs. Patients were deemed ineligible for the study if they fulfilled any of the following criteria: intraoperative removal of the Intellijoint HIP smart tool prior to measurements being taken with trial components which matched the final implant geometry; instability in the native operative hip such that the attending surgeon was unable to reproduce the radiographic position during baseline and follow-up measurements; inability to properly reproduce and/or identify radiographic measurement landmarks on pre and post-surgical radiographs; and, radiographs unable to be properly scaled in pre and post-operative analysis.
Intellijoint HIP Smart Tool
The Intellijoint HIP smart tool is an intraoperative digital guidance tool, used to assist orthopaedic surgeons in the assessment of leg length and offset changes during total hip arthroplasty (Fig. 1). The smart tool relies on miniature/optical infrared technology and integrated microelectronics to provide real-time intraoperative tracking of femoral leg length. The system incorporates pelvic and femoral platforms, fixed via bone screws to the ipsilateral iliac crest and greater trochanter, respectively. A camera, magnetically attached to the pelvic platform, and a tracker, magnetically attached to the femoral platform, relay data to laptop-based control software. During use, the workstation computes the positional relationship between the camera and the tracker and provides this information in real-time on the display.
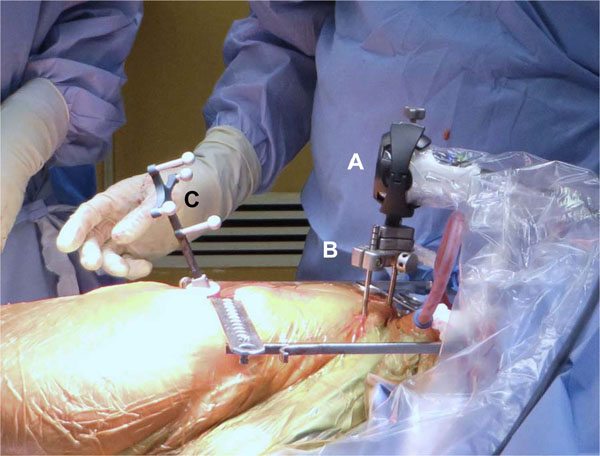
Surgical Procedure
Hip arthroplasty was performed using standard posterolateral approach techniques. No alterations to the surgical workflow are required and surgeons are able to integrate the smart tool into their workflow with minimal training. Intraoperatively, the Intellijoint HIP camera is coupled to the pelvis via a pelvic platform, which consists of two bone screws and a camera attachment mechanism. The bone screws are attached to the ipsilateral iliac crest or the superior acetabular dome. The tracker is coupled to the femur via a femur platform and attached to femur via a bone screw within the primary surgical wound.
The principle of operation of the Intellijoint HIP smart tool is as follows:
- Prior to hip dislocation, patient specific leg length and offset measurements of the native hip are captured using the camera and software,
- During trial reduction, a range of motion is conducted and tracker measurements are taken. This step is used to map the patient’s hip anatomy, and
- During trialing of the femoral stem, neck and head, measurements of leg length are provided to the surgeon on the display in real-time. Leg length data assist with the selection of correct implant size based on quantitative data and pre-operative planning.
Data Collection and Calculations
Data regarding final leg length was collected intraoperatively and stored by the Intellijoint HIP software. Patient records were reviewed for relevant patient history and to determine if any complications related to the use of the smart tool were reported. Post-operative radiographs were scaled using the known diameter of the head implant. Pre-operative radiographs were not scaled due to a lack of a suitable scaling object.
Two independent observers trained in radiographic assessment and blinded to the smart tool measurements performed the leg length calculations from the AP radiographs. Each observer was a trained orthopaedic surgeon with experience performing total hip arthroplasty using the posterolateral approach. Changes in leg length were calculated using the perpendicular distance between the inter-teardrop line and the most prominent aspect of the ipsilateral lesser trochanter [32]. The difference between the pre-operative and post-operative leg length for each patient was calculated by each observer and then averaged to provide the final leg length change. An established software program (MATLAB, Mathworks, Natick, MA) was used to select radiographic landmarks and scale images. Pre and post-operative radiographs were corrected for abduction in order to minimize the artifact.
Statistical Analysis
Alpha was set a priori at 0.05 for all statistical comparisons. Means were compared using independent samples t-tests and/or single-factor ANOVA. All mean values are presented as mean [95% confidence interval] or mean (standard deviation). Intra-observer validity was assessed via the intraclass correlation (ICC) [33]. An independent statistician uninvolved in the radiographic analysis or surgical procedures completed the statistical analysis.
Comparisons between radiographic and smart tool measurements of leg length were made using both Pearson’s r and the Bland-Altman technique [34, 35]. The Bland-Altman analysis provides a validated method for evaluating agreement between two methods of measurement when neither method is considered the “gold standard”. It is designed to determine the level of agreement between two methods of measuring a clinical outcome. The resulting Bland-Altman plot allows for determination of bias between mean differences of two methods of measurement and creates an agreement interval (the “statistical limit”), within which 95% of the difference of one method, as compared with a second method, falls [36]. A limit for clinically relevant leg length discrepancy (the “clinical limit”) was also included in the Bland-Altman plots. For the purposes of this analysis, this limit was set at ±5 mm to reflect the current clinical expectation [18, 37].
RESULTS
Patient Population
From an initial cohort of 31 potentially eligible patients, 25 were eligible for inclusion in this study. Of the six patients excluded from the study, 3 did not have reproducible landmarks on radiographs, 2 patients had poor bone quality that precluded the use of the Intellijoint HIP smart tool and 1 patient did not return for post-operative radiographs.
The mean age of included patients was 64.3 years (SD: 11.3, range: 44-85). Females comprised 50% (11/22 recorded) of patients. Mean body mass index (BMI) was 31.5 (SD: 7.2, range: 23.4-42.8). Mean procedural time was 76.2 mins (SD: 12.3, range: 59-98 mins). Demographic data are summarized in Table I.
Item | Result |
---|---|
Patient demographics Age, years (mean (SD)) BMI (mean, (SD)) Female, n/N1 (%) Male, n/N (%) |
64.3 (11.3) 31.5 (7.2) 11/22 (50) 11/22 (50) |
Surgery type, n/N (%) Primary Revision Surgery location, n/N (%) Left Right |
24/25 (96) 1/25 (4) 11/25 (44) 14/25 (56) |
Indication, n/N2 (%) Osteoarthritis Avascular necrosis Post-traumatic arthritis |
21/23 (91) 1/23 (4.5) 1/23 (4.5) |
Procedural time, minutes (mean, SD) | 76.2 (12.3) |
There was strong inter-rater agreement regarding leg length measurements calculated from radiographs (ICC: 0.83).
Leg Length Measurement
The mean difference between radiographic measurement and smart tool assessment of leg length was 1.3 mm [95% CI: -0.1 to 2.7]. A strong positive correlation was noted between leg length measurements calculated intraoperatively with the smart tool and radiographically (r=0.76) (Fig. 2A). There was no statistically significant difference between the mean leg length measurements as determined by radiographic and smart tool measurements (radiograph: 6.4 mm [4.3, 8.5] vs. smart tool: 7.7 mm [5.8, 9.6], p=0.41). The smart tool leg length measurement was within 5 mm of the radiographic measurement in 22/25 (88%) cases and within 10 mm of the radiographic measurement in 25/25 (100%) of cases.
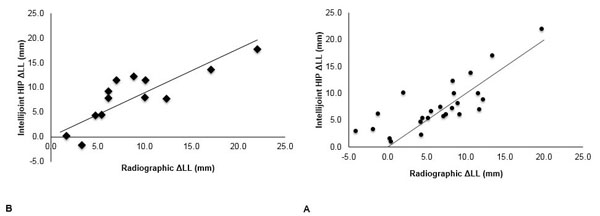
Placement of the pelvic platform varied within the cohort, due to the learning curve associated with the surgeons’ use of the smart tool. The initial 12 cases were performed with the platform fixed to the supra-acetabular region; the subsequent 13 were performed with the platform attached to the iliac crest. A post-hoc sensitivity analysis indicated that with the platform fixed to the iliac crest, the mean difference between the smart tool measurements and the radiographic measurements decreased to 0.6 mm (-0.6, 1.9) and the correlation between measurements improved to 0.82 (Fig. 2B). With the pelvic platform fixed to the iliac crest, 100% (13/13) of leg length differences were less than 5 mm.
A Bland-Altman analysis of the radiographic and smart tool methods of measuring leg length demonstrated excellent agreement. When all 25 eligible patients were included in the analysis, 96% (24/25) of measurements fell within the statistical limits of acceptability, while 84% (21/25) of measurements fell within the clinically acceptable range of ±5 mm (Fig. 3A). In patients where the pelvic platform was attached to the iliac crest, 100% (13/13) of measurements fell within both the statistical and clinical limits of acceptability (Fig. 3B).
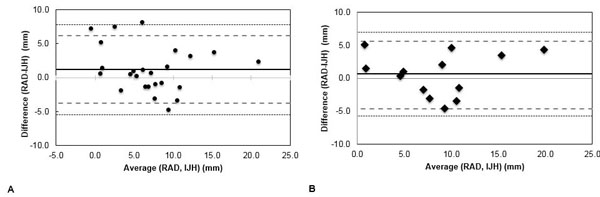
DISCUSSION
Leg length discrepancies following total hip arthroplasty can have significant consequences, substantially impacting patient quality of life and increasing the risk of medical malpractice litigation. Current intraoperative methods of monitoring leg length changes rely on either manual techniques such as tissue tensioning or mechanical outriggers, or advanced surgical navigation systems that, while accurate, are both expensive and cumbersome. The Intellijoint HIP smart tool is an intraoperative guidance tool providing accurate and precise measurements of leg length that was recently evaluated in a cohort of 25 patients undergoing total hip arthroplasty. The smart tool successfully provided accurate intraoperative measurements with no device-related or procedure-related adverse events reported.
The gait alterations associated with LLD following THA result in compensatory changes in hip geometry and altered gait biomechanics [38], leading to low back pain [8] and potential neurological deficits [9-11], among other consequences. Current manual methods of managing LLD intraoperatively are based on landmarking and crude measurements, and are susceptible to slight variations in patient position during surgery [18]. Femoral abduction as minor as 5 degrees has been noted to induce leg length errors of up to 8 mm [19]. Additionally, a recent study found that 74% of THA patients with a LLD of greater than 5 mm perceived that difference post-operatively, with the majority of those patients reporting significantly worse pain and Oxford scores, as compared with patients who were unaware of a LLD and/or those with a LLD less than 5 mm [39].
Pre-operative planning offers an opportunity for careful measurement prior to entering the surgical suite; however, intraoperative measurement remains the preferred method of managing leg length. Even crude intraoperative measurements have been shown to be more successful than pre-operative planning at minimizing LLDs, with one recent study observing that simple ruler measurement of leg length intraoperatively was associated with a smaller LLD (2±2 mm) than pre-operative planning (7±4 mm) [40]. Computer-assisted navigation, while providing improved intraoperative accuracy, requires multiple landmarking steps and significant pre-operative planning. The resulting increase in procedural time [23], pre-operative planning time and the cumbersome nature of navigation have limited its use to only 1-3% of orthopaedic procedures [30, 31].
The limit for acceptable leg length discrepancy following THA has been suggested at 10 mm [41-43], although differences of 5 mm represent better accuracy and are generally targeted by surgeons [18, 38]. The smart tool utilized in this study provided accurate data regarding pre and post-operative leg length, measuring leg length to within 5 mm of radiographic values in 88% of patients. Importantly these findings were achieved with a device that is no more intrusive or disruptive to the surgical workflow than any of the manual methods traditionally utilized. Indeed, in our study, the average procedure time was 76 minutes, which compares favourably with procedures utilizing mechanical measurement techniques, which range in duration from 73 [23, 44] to 104 minutes [45]. Conversely, computer-assisted navigation is associated with significant increases in procedural time, reported between 12 [46, 47] and 23 added minutes [23, 44, 45, 48].
Increased surgical time is associated with increased cost, and navigation systems are known to increase procedural costs, due to both the capital costs associated with the systems [26-28] and the per-procedure costs [27, 29]. At a cost of between $500-995 USD per case, and with no initial capital costs, the smart tool offers accuracy rivalling that of navigation systems at a substantially lower cost. The combined improvement in procedural time-add and the lower costs associated with the Intellijoint system suggest that it is a cost-effective option for THA. Robust cost-effectiveness studies are necessary to evaluate the potential cost savings associated with this system.
LIMITATIONS
This study has limitations. The retrospective nature of the study design and the lack of a control group decrease the veracity with which the conclusions can be extrapolated. The retrospective nature of the study also necessitated the use of standard radiographs to measure leg length. As such, no study-specific radiographic protocol was utilized. Also, pelvic radiographs, due to their 2-dimensional nature, are known to be susceptible to errors due to pelvic orientation, magnification, femoral abduction and femoral rotation [19, 49, 50]. Additionally, the pre-operative radiographs did not utilize a scaling object in the image. As a result, observers were required to assume that both pre and post-operative radiographs were to the same scale. These difficulties notwithstanding, AP radiographs are part of the standard of care for THA, as is their use for measurement of leg length and offset. The results of this study therefore mirror those of other studies in this field. To minimize the artifact associated with radiographs, future prospective studies will utilize strict radiographic protocols and pre and post-surgical scaling objects to minimize such errors.
CONCLUSION
The Intellijoint HIP smart tool accurately measured leg length intraoperatively, without significantly altering surgical workflow, increasing procedure time or requiring large capital investments prior to its use. This novel technology represents a safe and effective method of providing real-time data to surgeons and minimizing the effects of leg length discrepancies following total hip arthroplasty. Future studies will compare the smart tool prospectively to current intraoperative methods and will include robust investigations into the cost-effectiveness of the smart tool, in comparison to current manual and navigation methods.
LIST OF ABBREVIATIONS
ΔLL | = Change in leg length |
ANOVA | = Analysis of variance |
AP | = Anterior-posterior |
BMI | = Body mass index |
CI | = Confidence interval |
ICC | = Intraclass correlation |
IJH | = Intellijoint HIP |
LLD | = Leg length discrepancy |
OR | = Operating room |
RAD | = Radiographic measurement |
SD | = Standard deviation |
THA | = Total hip arthroplasty |
USD | = United States dollar |
DISCLOSURES
Dr. Grosso and Snider are paid consultants for Intellijoint Surgical. Dr. Grosso is an investor in Intellijoint Surgical. Dr. Muir is an employee of Intellijoint Surgical.
CONFLICT OF INTEREST
The authors confirm that this article content has no conflict of interest.
ACKNOWLEDGEMENTS
Declared none.