All published articles of this journal are available on ScienceDirect.
Periosteal Augmentation of Allograft Bone and its Effect on Implant Fixation - An Experimental Study on 12 Dogs§
Abstract
Purpose:
Periosteum provides essential cellular and biological components necessary for fracture healing and bone repair. We hypothesized that augmenting allograft bone by adding fragmented autologous periosteum would improve fixation of grafted implants.
Methods:
In each of twelve dogs, we implanted two unloaded cylindrical (10 mm x 6 mm) titanium implants into the distal femur. The implants were surrounded by a 2.5-mm gap into which morselized allograft bone with or without addition of fragmented autologous periosteum was impacted. After four weeks, the animals were euthanized and the implants were evaluated by histomorphometric analysis and mechanical push-out test.
Results:
Although less new bone was found on the implant surface and increased volume of fibrous tissue was present in the gap around the implant, no difference was found between treatment groups regarding the mechanical parameters. Increased new bone formation was observed in the immediate vicinity of the periosteum fragments within the bone graft.
Conclusion:
The method for periosteal augmentation used in this study did not alter the mechanical fixation although osseointegration was impaired. The observed activity of new bone formation at the boundary of the periosteum fragments may indicate maintained bone stimulating properties of the transplanted cambium layer. Augmenting the bone graft by smaller fragments of periosteum, isolated cambium layer tissue or cultured periosteal cells could be studied in the future.
INTRODUCTION
Due to insufficient bone stock, extensive bone grafting is often required in revision of a failed arthroplasty. Impacted morselized allograft bone is a well-established method for improving bone-stock and thereby achieving sufficient stability and anchorage of the revision implants [1-3]. However, it has been shown that the incorporation of bone graft into the host bone is not always complete [4, 5]. This may be a contributing reason for the higher failure rates and poorer functional outcome among patients following revision surgery [6]. In addition, several clinical trials have shown increased early implant subsidence when using impaction grafting [7, 8]. Autograft bone is often regarded as the gold standard of bone grafts, but for the large quantities often needed in revision joint arthroplasty, this is not a realistic option. It has been proposed, that the difference in osseointegration could be due to the difference in the cellular component in the graft material [9]. Whereas the cells in autograft bone are vital, freezing has largely destroyed the cellular components of allograft bone.
Periosteum covers nearly every bone in the body and plays a major role in promoting bone growth and bone repair [10]. It consists of two distinct layers, an outer fibrous layer and an inner cambium layer that has significant osteoblastic potential [11]. The cambium contains mesenchymal progenitor cells, osteoblasts and fibroblasts [12] and provides essential cellular and biological components necessary for fracture healing and bone repair [13, 14].
The objective of this experiment is to investigate whether the bioactivity of allograft bone can be augmented by adding autologous tissue containing bone-competent cells. Specifically, we add fragmented, freshly harvested autologous periosteum, as this is often readily available during surgery and contains pluripotent cells with the potential of differentiation into bone-forming cells [15-18]. By enhancing the bioactivity of the bone graft, we hope to achieve a more complete and better initial bone graft incorporation, which may aid in increasing the longevity of a revision implant. Therefore, we hypothesized that augmenting the allograft bone by adding fragmented autologous periosteum would improve fixation of grafted implants (mechanically and histomorphometrically).
MATERIALS AND METHODOLOGY
We conducted a paired randomized controlled study in twelve skeletally mature female American hounds with a mean weight of 24 kg (22– 26 kg) and mean age of 16 months (12-21 months). Each animal received a total of two porous-coated titanium implants in the metaphyseal cancellous bone of the distal femur (Fig. 1). All implants were surrounded by a 2.5 mm concentric gap into which allograft bone, with or without addition of fragmented periosteum, was impacted. The implantation site (left or right limb and medial/lateral epicondyle) of each treatment group was alternated systematically with random start. The observation period was four weeks.
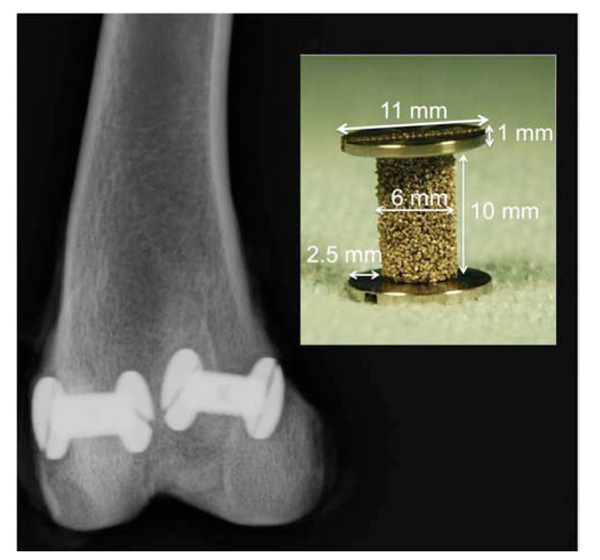
Gap-implant in distal femur. Radiograph taken post mortem. The implant with dimensions is shown.
Sample-size was determined prior to the experiment and was based on previous studies using the same allograft implant model having a coefficient of variance of 35%. The minimal clinically relevant difference to be detected in this study was set to 30%. Two-sided α and β were set to 5% and 20%, respectively. Two extra animals were added to the calculated sample size of ten to counteract a decrease in power if implants from one or two animals were lost to follow-up and subsequent analysis
The animals were raised for scientific purposes and were housed individually but socialized in groups with two hours of daily exercise. The animal experiment was approved and monitored by the Institutional Animal Care and Use Committee (Minneapolis Medical Research Foundation (MMRF), Minneapolis, MN, submission ID 08-08-02, protocol number 05-01). Surgery and observation were performed at the Animal Care facilities of the Minneapolis Medical Research Foundation at the Hennepin County Medical Center (Minneapolis, MN), according to the regulations of the National Institute of Health. Preparation of the bone samples, mechanical testing and histomorphometry were carried out at the Orthopaedic Research Laboratory of Aarhus University Hospital, Denmark.
Implants
For the experiment, we used 24 porous-coated titanium alloy (Ti-6A1-4V) implants manufactured by DePuy Inc. (Warsaw, IN). The implants were custom-made, cylindrical, 6 mm in diameter, 10 mm in height (Fig. 1). An end plate with a diameter of 11 mm was attached to one end of the implant and inserted into an 11-mm pre-drilled bone-defect. The end plate centered the implant, providing a uniform circumferential 2.5-mm peri-implant gap. After impaction of the variously prepared allograft, the gap was closed with another 11-mm end plate, which ensured implant stability and bone graft containment.
Bone Graft
Bone graft was harvested immediately post mortem under sterile conditions from two dogs not included in the study. The proximal humerus, the proximal tibia and the distal femur were used. After removal of all soft tissues and cartilage, the remaining bone was morselized using a standard bone mill (Biomet®, Warsaw, Indiana, USA) on fine setting, creating bone chips of 1-3 mm. The bone graft from the two dogs was mixed together and divided into 24 tightly compressed portions of 1 ml and stored in sterile double-containers at –80°C. The preparation of graft for the whole study was undertaken in one setting.
Surgery
Under general anaesthesia and sterile conditions, the femoral epicondyles were exposed by a medial and lateral incision. The joint capsule was opened and the collateral ligament identified. A cylindrical bone defect with a depth of 12 mm was drilled over a 2.5 mm guide wire using an 11-mm cannulated drill bit. To avoid thermal damage, a drill speed of a maximum of two rotations per second was used. To ensure uniform central placement, the implants were inserted with a specially designed hollow cylinder impaction tool with an inner diameter of 7 mm and an outer diameter of 11 mm. The same tool, which could slide over the implant, was used to impact the bone graft into the peri-implant gap. A circular periosteal flap of 1 cm in diameter was elevated from the anterior medial surface of the proximal tibia and fragmented with a scalpel (Fig. 2). This created small (< 1mm2) fragments of periosteum, which was hereafter immediately mixed together with bone graft and impacted around the implant. Finally, the end plate was mounted, and the soft tissues closed in layers. Bupivacaine, 0.5% was delivered locally following closure. Prophylactic antibiotics were administered as follows. A 1g dose of Ceftriaxone was given intravenously to each animal before surgery. Post-operatively, the animals were given Ceftriaxone 1g intramuscularly for three days. A Fentanyl transdermal patch (75 μg/h) lasting three days was given as postoperative analgesic treatment. After surgery, the animals were allowed unlimited activity
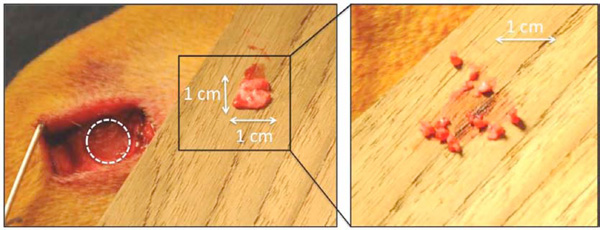
Left: Periosteum harvest from the anterior medial surface of the proximal tibia. Right: Enlarged picture of the fragmented periosteum.
Specimen Preparation
After the observation period of four weeks, the animals received sedation with Acepromazine, 0.5 mg/kg, anaesthetized using Propofol, 4 mg/kg, and euthanized with an overdose of hypersaturated barbiturate. The distal part of the femurs was dissected and immediately frozen and stored at -80°C. Preparation of the specimens was performed blinded. Prior to dividing the bone into the mechanical and histomorphometric sections, the distal femora were thawed for one hour and the outermost 1 mm of the implant-bone sample below the 11-mm end plate was cut off and discarded. Two bone-implant sections were cut perpendicular to the long axis of each implant, using a water-cooled diamond band saw (Exakt-Cutting Grinding System, Exakt Apparatebau, Norderstedt, Germany). The inner 5.5 mm section of the implant was processed for histomorphometric evaluation by dehydration in graded ethanol (70– 100%) and embedded in methylmethacrylate (Art. 800590, Merck, Darmstadt, Germany) and the outer 3.5-mm section was stored at -20°C and used for mechanical push-out testing.
To ensure uniformly randomized sectioning and thereby optimizing the stereological analysis, the embedded section was randomly rotated around its long axis before sectioning. Using vertical sectioning technique [19], four 30-μm-thick central histological sections were cut parallel to the implant axis. These techniques provide highly reliable results with negligible bias [20]. A hard-tissue microtome (KDG-95; MeProTech, Heerhugowaard, The Netherlands) was used to cut the sections and they were surface stained with 0.1% Toluidine Blue (pH=7) (Fluka, Sigma-Aldrich®, St. Louis, MO, USA) for 10-min, rinsed and mounted on glass. This preparation provided blue staining of non-calcified tissue and light blue staining of calcified tissue. The different types of calcified tissues such as newly formed bone (woven bone) and bone graft (lamellar bone) were categorized on the basis of their morphological characteristics [21].
Mechanical Testing
The thawed specimens were tested to failure by axial push-out test on an MTS Bionics Test Machine (MTS 858 Mini Bionix, Software MTS Test Star 790.00 Version 4.0C) (Fig. 3). The testing was performed blinded and in one session. The specimens were placed with the cortical side facing up on a metal support jig with the 6-mm diameter implant centered over a 7.4-mm opening and under a cylindrical test probe of 5-mm diameter. A preload of 2N defined the contact position at the start of the test. The implant was pushed from the surrounding bone in the direction of the implant axis at a velocity of 5 mm/min. Load (N) versus implant displacement (mm) data were continuously recorded. From these data, the mechanical parameters: The ultimate shear strength (MPa), Apparent shear stiffness (MPa/mm) and Total energy absorption (KJ/m2) were calculated as earlier described [22].
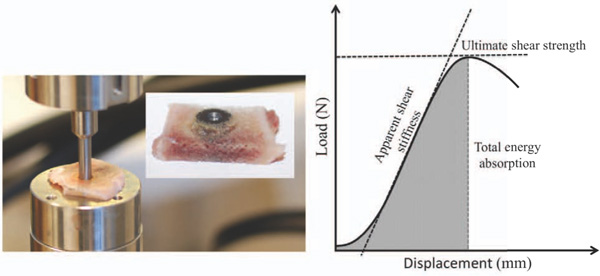
Mechanical testing. Left: Push-out test performed with the 3.5 mm thick specimen placed on metal support jig. In the corner, a picture of the implant/bone specimen after the implant has been pushed from the bone, demonstrating in-substance bone failure mode. Displacement velocity 5 mm/min. Right: Load (N) displacement (mm) curve enables calculation of ultimate shear strength (MPa), apparent shear stiffness (MPa/mm), and total energy absorption (KJ/m2)
Blinded histomorphometry was performed using stereological software (newCAST-version 3.4.1.0; Visiopharm A/S, Horsholm, Denmark). We quantified the area fractions of new bone, fibrous tissue and graft bone in contact with the surface of the implant and the volume fractions of these tissues in the gap around it (Fig. 4). Tissue ongrowth was defined as tissue directly at the implant surface and estimated by a line intercept technique [19]. Volume of tissue fractions in the gap were estimated using point counting technique [23].
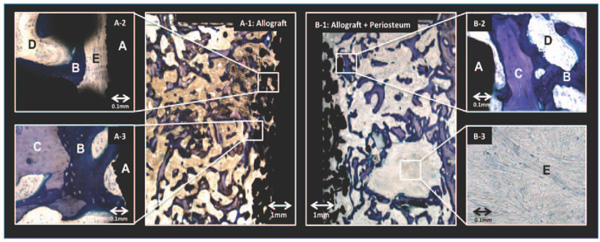
Histology: Representative histologic sections of the control group (A: Allograft) and the intervention group (B: Allograft + periosteum). The sections are from two implants inserted in the same animal. Left side (allograft only): A-1: Overview of implant and bone graft; A-2: Thin fibrous tissue membrane in contact with implant; A-3: Allograft bone chip with ongrowth of new bone in contact with implant. Right side (Allograft + periosteum): B-1: Overview of implant and bone graft. Large island of fibrous tissue is seen in the gap with activity of new bone formation in its vicinity. B-2: Allograft bone chip with ongrowth of new bone in contact with implant; B-3: Magnification of the island revealing solid fibrous tissue. A = Implant, B = New bone, C = Allograft bone, D = Marrow space, E = Fibrous tissue. (Stain, toluidine blue; magnification, x28 (A-1 and B-1), x230 (A-2, A-3, B-2 and B-3).
Statistical Analysis
All mechanical data followed a normal distribution as evaluated by q-norm plots of the residuals and Student’s paired t-test was used to evaluate differences between treatment groups. The mechanical data are presented as means with standard deviation and the absolute differences between treatment groups as means with 95% confidence intervals (CIs). Data from the histomorphometrical assessment were normally distributed for area fraction of new bone, and volume fraction of new bone and bone graft. Differences between treatment groups were determined using Students t-test. The area fraction of bone graft and fibrous tissue, and the volume fraction of fibrous tissue were not normally distributed manly due to many values close to zero and difference between treatment groups were determined using Wilcoxon sign-rank test. Estimates are presented as medians with inter-quartile ranges (IQR). Differences between medians or means were considered statistically significant for p-values less than 0.05. The analysis was performed using STATA statistical software (Stata 11.2, StataCorp, College Station, Texas).
RESULTS
All twelve animals were fully weight bearing within three days after surgery. Eleven animals completed the observation period without any signs of infection or other complications. One animal died five days prior to completion of the four-week observation period. An autopsy was performed, and the cause of death was determined to result from intestinal invagination and not the surgery performed. The animal had been in normal health until that time and as the observation period almost completed the animal was not excluded, and the bones were harvested and included in the analysis.
Mechanical Results
Periosteal augmentation of allograft bone revealed no statistically significant difference between treatment groups regarding the three mechanical parameters: Ultimate shear strength (p=0.79), Apparent shear stiffness (p=0.83) and Total energy absorption (p=0.62) (Fig. 5).
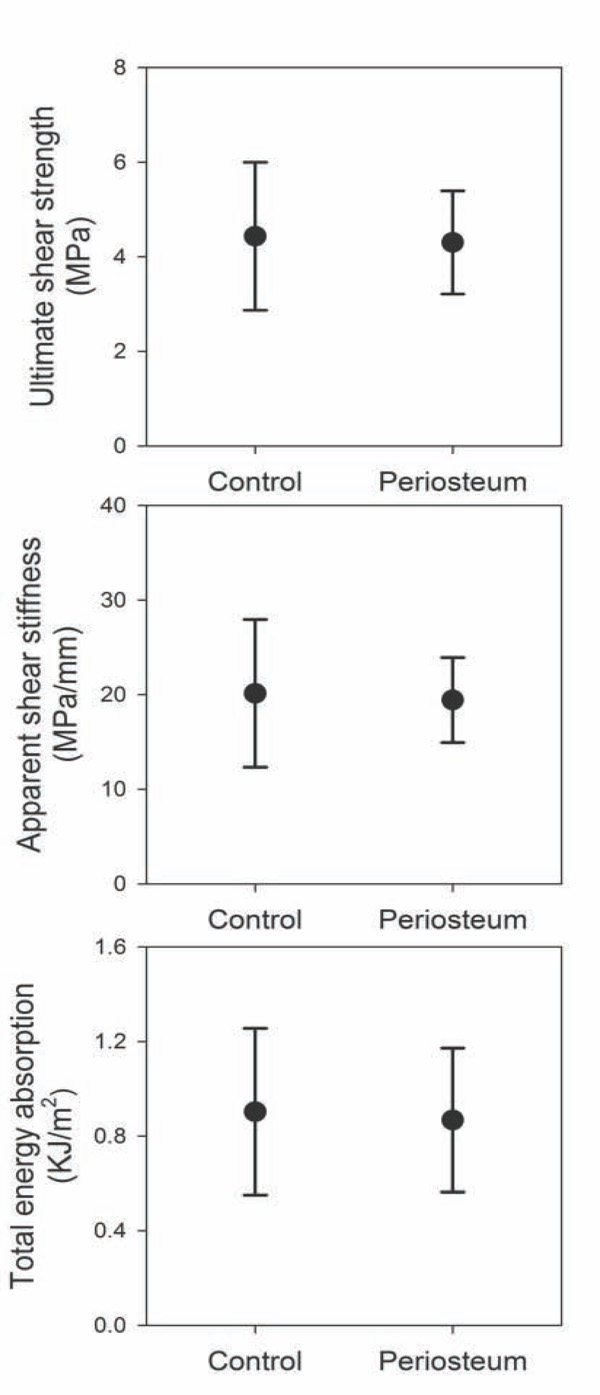
Mechanical testing - Scatter plots showing results from mechanical push-out test. Ultimate shear stiffness, apparent shear strength, and total energy absorption; n(control) = 12, n(periosteum) = 12; [mean (95% CI)].
Histological Results
Histology: New bone and graft bone filled the gap in both the control and periosteum groups. A common finding in the periosteum group was islands of fibrous tissue with high activity of new-bone-formation in its vicinty (Fig. 4). It could not be determined to which extent these islands were newly formed or were remnants of the implanted periosteum.
Histomorphometry
Compared to the untreated allograft controls, the periosteum-augmented implants had an 18% reduction in ongrowth of new-bone (Table 1a) while no difference was observed in new bone formation in the gap around the implant. The volume fraction of fibrous tissue was increased in the periosteum group and increased fibrous tissue ongrowth were observed, although not statistically significant (p=0,12) (Table 1b).
Histomorphometrical Ongrowth: Tissue Area Fractions on Implant Surface
Surface Fraction | New Bone Mean % (SD) | Bone Graft Median % (IQR) | Fibrous Tissue Median % (IQR) |
---|---|---|---|
|
|||
Control | 17 (6.3) | 0.0 (0.0 - 0.1) | 0.7 (0.0 - 1.9) |
Periosteum | 14 (3.6) | 0.0 (0-0 - 0.0) | 3.8 (0.5 - 5.0) |
|
|||
Periosteum-Control | 3 (0.0; 6.0) | ||
Students t-test | p = 0.05 | ||
Wilcoxon | p = 0.13 | p = 0.12 |
New bone is presented as mean with standard deviation (SD) and the absolut difference between treatment groups as mean difference with 95% confidence interval (CI). Bone graft and fibrous tissue are presented as median with interquartile range (IQ).
Histomorphometrical Ingrowth: Tissue Volume Fractions in the Gap
Volume Fraction | New Bone Mean % (SD) | Bone Graft Mean % (SD) | Fibrous Tissue Median % (IQR) |
---|---|---|---|
|
|||
Control | 21 (3.7) | 8.4 (3.3) | 0.1 (0.0 - 0.2) |
Periosteum | 18 (4.0) | 6.7 (2.5) | 4.4 (0.3 - 6.4) |
Periosteum-Control | -2.3 (-5.2; 0.6) | -1.7 (-3.6; 0.2) | |
|
|||
Students t-test | p = 0.11 | p = 0.07 | |
Wilcoxon | p = <0.01 |
New bone and bone graft are presented as mean with standard deviation (SD) and the absolut difference between treatment groups as mean difference with 95% confidence interval (CI). Fibrous tissue is presented as median with interquartile range (IQ).
DISCUSSION
Impacted allograft bone can provide sufficient initial mechanical support of a revision implant. But, to maintain good long-term implant fixation, adequate new bone formation is needed. The preparation of bone graft by freezing or radiation largely destroys its vital cellular components, rendering it with reduced osteogenic capacity [9]. This may contribute to incomplete bone graft incorporation, which has been described as a common finding around grafted implants [4, 5]. Periosteum plays a major role in promoting bone growth and bone repair [10, 13, 14] and contains multiple cells with osteoblastic potential [11, 15-18]. Augmenting the bone graft with fragmented periosteum may provide a signal for improved osteogenic differentiation, and possibly add pluripotent mesenchymal progenitor cells for facilitating initial bone healing.
Our experimental model was designed to study early fixation and osseointegration of an uncemented implant component surrounded by a bed of impacted bone graft [24]. It was simple and had a high degree of control of parameters [25], but the model had limitations. The implant model is not subject to several clinically relevant influences such as joint fluid pressure and direct load transmission, and the results are therefore limited, as the full effects of weight-bearing conditions are not addressed. Furthermore, the grafted defect was created in healthy bone of young animals, and not the compromised bone typically surrounding a loose implant in humans. Dogs were chosen as experimental animals because their bone quality and trabecular density reflect human bone well [26]. However, it is noted that the bones of dogs remodel three times more quickly than human bone [27]. The observation time of four weeks was chosen since the aim was to study influence of periosteal augmentation on early implant fixation. Early implant subsidence has been shown to be associated with late implant loosening [28, 29] and it is therefore of interest to study any experimental approach at an early time point. The method used for periosteum harvest could in some animals have been incomplete causing incomplete harvest of the inner cambium layer. This may explain why some specimens only showed limited new bone activity in the vicinity of the fibrous tissue islands. The ability for the surgeon to conduct a complete periosteal harvest was not evaluated. The model allows paired comparison of two treatment groups, by which the influence of biological differences between the animals was reduced.
Contrary to our hypothesis, we found no benefit of adding periosteum to the allograft bone. Overall, with periosteum we found a reduced osseointegration but unaltered mechanical properties. Histologically, several implants in the periosteum group showed large fibrous tissue islands in the peri implanteric gap. High activity of new bone formation in the vicinity of these islands was a common finding (Fig. 4). This may indicate maintained osteoinductive properties of the cambium layer, which is in accordance with earlier studies showing bone formation on transplanted autologous periosteum [17, 18].
The osteoinductive mechanism of fragmented periosteum may be multifactorial. By fragmenting the periosteum, the cambium layer maintains its relation to the original periosteum tissue. This might extend its effect on new-bone formation in the non-vascularized impacted environment. An additional mechanism is the potential liberation of essential bone stimulating substances once the cambium cells necrotize. Therefore, augmenting the bone graft by smaller fragments of periosteum, isolated cambium layer tissue or cultured periosteal cells would be interesting approaches for future research in grafted implants.
CONCLUSION
This study evaluated a simple method for heightening the bioactivity of morselized allograft bone in a one-stage procedure. We were not able to improve the mechanical fixation but the results showed potential histological benefits in terms of enhanced new-bone formation around the periosteum fragments.
CONTRIBUTIONS OF AUTHORS
Jeppe Barckman (JBA): design, surgery, evaluation, statistics, manuscript, discussion.
Jorgen Baas, Mette Sorensen, Joan E. Bechtold: design, surgery, manuscript comments, discussion.
Kjeld Soballe: design, manuscript comments, discussion.
CONFLICT OF INTEREST
DePuy Inc. kindly donated all the implants unconditionally.
National Institutes of Health (NIH) (AR-42051) and The Danish Rheumatism Association (R101-A1968) supported this study.
ETHICAL APPROVAL
Each author certifies that his or her institution approved the animal protocol for this investigation and that all investigations were conducted in conformity with ethical principles of research.
NOTES
§ This work was performed at the Orthopaedic Research Laboratory, Aarhus University Hospital, Aarhus, Denmark (specimen preparation, testing, and analysis), and Orthopaedic Biomechanics Laboratory, Midwest Research Foundation, Minneapolis, MN, USA (surgery).
ACKNOWLEDGEMENTS
The authors wish to thank laboratory technician Jane Pauli, Orthopaedic Research Laboratory, Aarhus University Hospital for technical assistance with histological sections and Orthopaedic Biomechanics Laboratory, Minneapolis, MN, USA, for surgical assistance. DePuy Inc., Warsaw, IN, USA kindly provided all base implants.
The authors would like to acknowledge funding for this study received from and NIH (AR 42051) and The Danish Rheumatism Association (R101-A1968). The sponsors did not participate in the design of the study, in the evaluation of the results, or in writing of the article.