All published articles of this journal are available on ScienceDirect.
3D-Printed Patient-Specific ACL Femoral Tunnel Guide from MRI
Abstract
Background:
Traditional ACL reconstruction with non-anatomic techniques can demonstrate unsatisfactory long-term outcomes with regards instability and the degenerative knee changes observed with these results. Anatomic ACL reconstruction attempts to closely reproduce the patient's individual anatomic characteristics with the aim of restoring knee kinematics, in order to improve patient short and long-term outcomes. We designed an arthroscopic, patient-specific, ACL femoral tunnel guide to aid anatomical placement of the ACL graft within the femoral tunnel.
Methods:
The guide design was based on MRI scan of the subject's uninjured contralateral knee, identifying the femoral footprint and its anatomical position relative to the borders of the femoral articular cartilage. Image processing software was used to create a 3D computer aided design which was subsequently exported to a 3D-printing service.
Results:
Transparent acrylic based photopolymer, PA220 plastic and 316L stainless steel patient-specific ACL femoral tunnel guides were created; the models produced were accurate with no statistical difference in size and positioning of the center of the ACL femoral footprint guide to MRI (p=0.344, p=0.189, p=0.233 respectively). The guides aim to provide accurate marking of the starting point of the femoral tunnel in arthroscopic ACL reconstruction.
Conclusion:
This study serves as a proof of concept for the accurate creation of 3D-printed patient-specific guides for the anatomical placement of the femoral tunnel during ACL reconstruction.
1. INTRODUCTION
Anterior cruciate ligament (ACL) reconstruction has repeatedly demonstrated successful outcomes at short term follow-up. It aims to improve the stability of the knee, facilitate return to sports and may help prevent osteoarthritis produced in ACL deficient knees [1-3]. However, several studies can demonstrate unsatisfactory long-term outcomes, particularly in high level athletes, owing to the development of clinically symptomatic instability and a low rate of return to pre-injury sporting levels [4-9].
Traditional ACL reconstruction included transtibial drilling of the ACL femoral tunnel, with a focus on isometric graft placement and avoidance of notch impingement [10]. More recently, surgical techniques for creating the ACL femoral tunnel have been reconsidered, with a focus towards anatomical placement [11-15]. Anatomic ACL reconstruction can be defined as the functional restoration of the ACL to its native dimensions, collagen orientation and insertion sites [16]. Femoral tunnel anatomical positioning is achieved through marking of the tunnel via the anteromedial or an accessory anteromedial portal [12-16]. Retrograde reamers have also been introduced as a method to aid in placement of the femoral tunnel at the anatomical ACL position [17]. A primary focus towards anatomic reconstruction has been shown to better restore anterior translational as well as rotational stability to an ACL deficient knee [18-21].
The ACL ‘femoral footprint’ is described as the midpoint between the anteromedial and posterolateral ACL bundles - marking the midpoint of the native anatomical ACL [22]. The mean anatomic centrum of the ACL femoral footprint has been described radiologically as 43% of the distance from the proximal margin of the posterior condyle of the femur to the distal most aspect of the condyle, as viewed on a lateral radiograph of the lateral wall of the intercondylar notch [23]. Marking the femoral footprint through direct visualization arthroscopically has been described through identifying the lateral intercondylar ridge and marking a point half way between this and the inferior articular cartilage [24]. Whilst these measurements and landmarks provide a reference, they are not specific to any one patient’s anatomy. Non-anatomic ACL graft placement is the most common technical error leading to recurrent instability following ACL reconstruction [25, 26]. Mid-bundle techniques potentially have a higher graft re-rupture rate, however, this does not take into account truly anatomical placement of the graft in accordance with the patient’s native femoral footprint as identified on MRI. Patient specific ACL reconstruction has been proposed as a means to achieving a truly anatomical reconstruction [27].
Three-dimensional (3D) printed guides have been reported for various orthopaedic procedures, such as pelvic osteotomy [28], fixation for acetabular fracture [29], spinal instrumentation [28, 30], knee arthroplasty [31], hip arthroplasty [32] and corrective osteotomy of the upper extremity [33, 34].
Our aim was to design a 3D printed patient specific ACL femoral tunnel guide, based on magnetic resonance imaging (MRI) scan of the patient’s contralateral uninjured knee, for accurate intraoperative placement of the femoral tunnel within the ACL femoral footprint in single bundle ACL reconstruction to place the femoral tunnel in a truly anatomical position.
2. METHODS
A standard protocol MRI of a patient’s knee without ACL injury was carried out. The scanners used were 1.5 Tesla Siemens scanners. We used a MRI protocol for fat-saturated proton density images (PDFS) in 3 planes, coronal/axial/sagittal, and a sagittal T1 weighted image.
The images were transferred via DICOM files to a personal computer running OsiriX image processing software (Pixmeo, Geneva, Switzerland). Images were then subsequently analyzed for several anatomical landmarks: the patient’s native ACL femoral footprint (Fig. 1), the proximal and posterior edges (Fig. 2), and the distal edge (Fig. 3) of the articular cartilage on the lateral wall of the femoral notch. Distances were then calculated to determine the position of the center of the ACL footprint relative to the three articular cartilage points (Fig. 4). Three independent trained observers (orthopaedic surgeons) carried out three separate measurements for each anatomical landmark. The mean of the multiple measurements for each landmark was then used for subsequent analysis and 3D printing. Inter-observer variation was measured by means of intraclass correlation coefficient (ICC) with 95% confidence intervals (CI) using a random-effects model.
These measurements and points were then utilized to create a 3D computer aided design (CAD) model of a custom guide. This was done using the 3D CAD program 123Design (Autodesk Ltd., Farnbourgh, UK). The guides were designed with an entry point at the site of the ACL femoral footprint to allow access of a 3mm Chondro Pick (Arthrex inc., Naples, Florida) through the guide to mark the starting point of the femoral tunnel. The 3D model was exported as an STL file suitable for 3D printing. The STL file was uploaded to an online 3D printing service and the physical guide was created in transparent acrylic based photopolymer, PA220 plastic and 316L stainless steel.
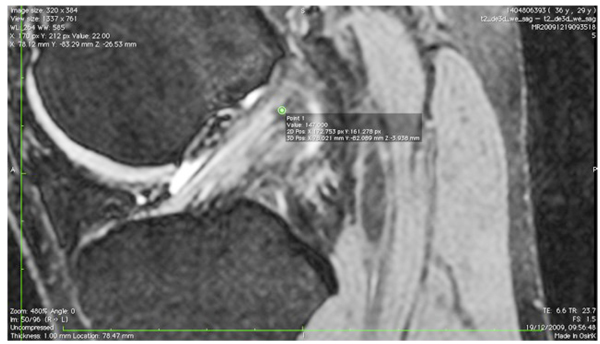
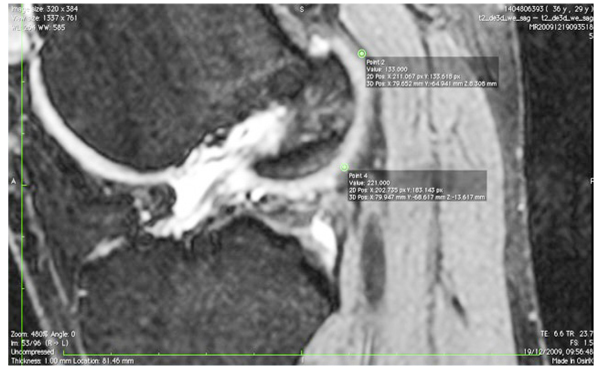
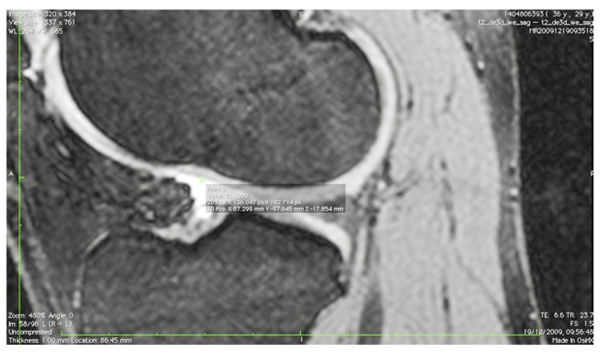
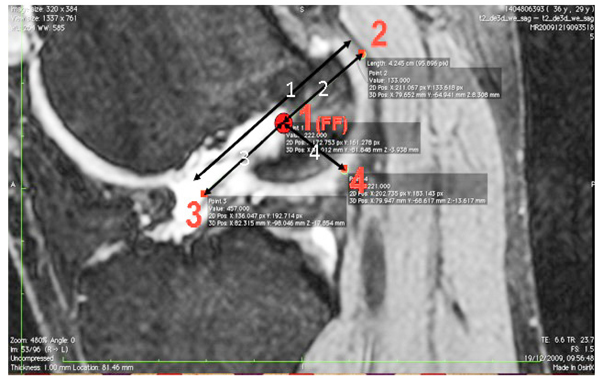
The models created were measured using vernier calipers (Mitutoyo 500-196-20 0-150 mm 6-inch Absolute Digimatic Caliper, Mitutoyo Corp., Japan). Three independent observers carried out three separate measurements of the models. The mean of the multiple measurements was compared to the original MRI dimensions and 3D CAD model (Graphpad Prism 6, Graphpad Inc. CA, USA). Paired student t test was performed to assess for statistical significance. Inter-observer variation was measured by means of ICC with 95% CI using a random effects model.
3. RESULTS
Three patient specific ACL femoral tunnel guides (transparent acrylic based photopolymer, PA220 plastic and 316L stainless steel) were created. (Fig. 5) Distances measured included proximal to distal articular cartilage, posterior articular cartilage to femoral footprint, distal articular cartilage to femoral footprint and proximal articular cartilage to femoral footprint. The models produced were accurate with no statistical difference in size and positioning of the center of the ACL femoral footprint, relative to the articular cartilage margins on the lateral wall of the femoral notch, when compared to the original CAD model and MRI scans (MRI/CAD Vs. PA220 p=0.3753, MRI/CAD Vs. 316L p=0.0683, MRI/CAD Vs. Photopolymer p=0.3450) (Table 1). Inter-observer variability analysis showed excellent correlation for both MRI landmark identification (ICC 1.00, CI 0.997 to 1.000) and guide measurement (ICC 1.00, CI 1.00 to 1.00). The costs for the 3D printed models were £3.50 for the PA220 plastic, £15 for the transparent photopolymer and £25 for the 316L stainless steel. The time taken from MRI to delivery for the physical models was 7 days.
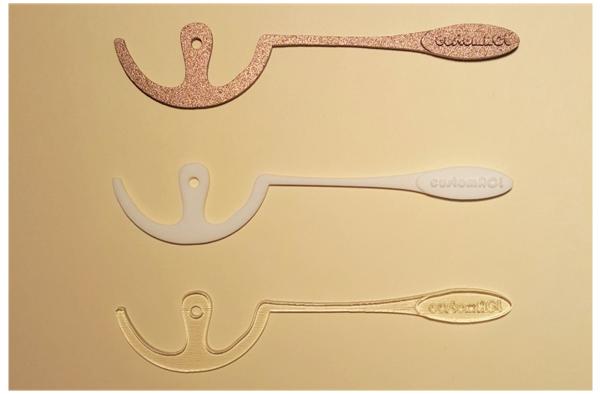
4. DISCUSSION
Our study demonstrates that a 3D printed patient-specific ACL femoral tunnel guide can be created, based on a MRI scan of the contralateral uninjured knee, with low cost and of short duration from conception to creation. The guide, via entry of the anterolateral portal, would allow the operating surgeon to mark out the starting point of the femoral tunnel with a 3mm Chondro Pick, via the entry point within the guide.
![]() |
---|
There is increasing evidence indicating that the anatomic ACL reconstruction produces greater restoration of anterior translational as well as rotational stability to an ACL deficient knee [18-21]. Anatomic reconstruction of the ACL should take into account the differences between the anatomical characteristics of each patient in order to potentially restore native ligament function, with known variation between individuals in the shape and size of the ACL [35]. A “one-size-fits-all” approach does not adequately reproduce the native ACL. Non-anatomical reconstruction procedures may eliminate anterior/posterior laxity, but fail to restore rotational stability [36, 37]. This has been investigated with In vivo kinematic studies, which showed that non-anatomic ACL reconstruction procedures fail to restore normal dynamic knee function. Georgoulis et al. examined ACL-deficient knees before and after non-anatomic ACL reconstruction, using video-motion analysis. ACL-deficient patients demonstrated greater tibial internal rotation during walking. This reached near normal levels following non-anatomical reconstruction. During higher demand activities however, such as stair descent and pivoting, tibial rotation was significantly larger in the non-anatomical ACL reconstructed knees compared to the contralateral intact ACL knee [38]. Brandsson et al. similarly found that tibial rotation was not restored with non-anatomical ACL reconstruction when measured using continuous radiostereometric analysis [39]. MRI investigation during static weightbearing showed that whilst non-anatomical ACL reconstruction reduced sagittal laxity of the knee to within normal limits, it did not restore the normal tibiofemoral kinematics [40]. More physically demanding activities have been investigated using high-speed radiographic imaging systems. Tashman et al. used a 250 frame/s dynamic stereo x-ray system to evaluate in vivo kinematic of the knee during downhill running in patients that had undergone non-anatomic ACL reconstruction. Anteroposterior translation was restored, but the reconstructed knees were more externally rotated and more adducted relative to the contralateral, uninjured knees. These rotational changes were associated with shifts in the areas of joint contact and a reduction in medial-compartment joint space during dynamic loading [41, 42]. Standard non-anatomical tunnels reproduce only a fraction of the native ACL. With respect to the femoral insertion, Hensler et al. showed that only 61% of the femoral insertion is reconstructed with standard tunnel preparation [43]. Abebe et al., using biplanar fluoroscopy and MRI, reported that anatomic femoral placement of the graft resulted in kinematics that more closely replicated that of the intact knee when compared to a non-anatomical femoral placement [44]. By individualizing ACL reconstruction, we may be able to reproduce more of the native anatomy and improve patient outcomes.
Articles regarding the creation of a 3D printed custom ACL guide from the patient’s contralateral knee do not feature in current literature. Where 3D printed patient specific guides have been used in other orthopedic procedures, favorable outcomes have been achieved. Reported advantages to patient-specific surgical guides include a reduction in operating times and improvement in the accuracy of surgical interventions, due to the guides’ personalization [45]. Hananouchi et al developed a 3D printed patient specific surgical guide for cup insertion in total hip arthroplasty. In their study, the mean absolute deviation from the patient’s preoperative planned alignment of the cup was 2.8° for abduction and 3.7° for anteversion [32]. A cadaveric study utilizing customized cutting jigs for total knee arthroplasty showed mean errors for alignment and bone resection within 1.7° and 0.8 mm respectively [30]. Whilst showing desirable results, the authors of these studies did not compare the results of customized guides to outcomes achieved without customized guides. Patient specific guides have increased popularity in spinal surgery owing to the reported improvement in accuracy of instrumentation [45]. Bundoc et al. developed a 3D printed patient specific drill guide for pedicle screw insertion into the subaxial cervical spine. They performed a cadaveric study on fifty pedicles to investigate the accuracy of screw placement. Their findings showed the patient specific guides to have an overall accuracy rate for cervical pedical screw placement of 94%, greater than the current reported gold standard (fluoroscopy-guided insertion) accuracy rates of 85-91% [46]
In the case of total knee arthroplasty, a recent meta-analysis has shown that patient specific guides provide no superior accuracy than using manual implementation during total knee arthroplasty [47]. There is no data regarding outcomes of patient-specific ACL guides currently within the literature.
It is hypothesized that the use of a patient specific guide would allow better identification of the ACL footprint than notch clearance and visualization of the ACL alone. A clear ACL footprint within the injured knee is not always available. Identification of the exact origins of the ACL in a traumatic knee as viewed through a 30-degree arthroscope is not always possible. The next step for this research is a cadaveric based study, utilizing the guides to carry out the creation of the ACL femoral tunnel and subsequent analysis of tunnel placement in relation to the contralateral knee.
The guides were easy to create and produce, taking only a week and with a cost of between £3.50 and £25. A modified MRI protocol scanning both knees of patients with suspected ACL injuries would allow identification of the native ACL femoral footprint at the same time of diagnosis of ACL injury, MRI of the contralateral knee utilizing only the 2 planes required for guide design incurs an additional ten minutes of MRI time. Following sterilization, these surgical guides could be used intraoperatively in any hospital.
This study serves as the first step and a proof of concept for the accurate creation of patient specific 3D printed guides for the anatomical placement of the femoral tunnel during ACL reconstruction.
ETHICS APPROVAL AND CONSENT TO PARTICIPATE
Ethics not required (measurements performed retrospectively on previously performed MRI), consent obtained.
HUMAN AND ANIMAL RIGHTS
Nothing to declare.
CONSENT FOR PUBLICATION
Consent obtained for publication.
CONFLICT OF INTEREST
The authors declare no conflict of interest, financial or otherwise.
ACKNOWLEDGEMENTS
No specific acknowledgments.