All published articles of this journal are available on ScienceDirect.
Three-Dimensional Computed Tomographic Analysis for Comminution of Pertrochanteric Femoral Fracture: Comminuted Anterior Cortex as a Predictor of Cutting Out
Abstract
Background:
Fixed angle sliding hip screw devices allow controlled impaction between the head neck fragment and the femoral shaft fragment in the surgical treatment of pertrochanteric fractures. This study was performed to evaluate the frequency and pattern of comminution at the fracture site, which may prevent the intended impaction.
Materials and Methods:
Three-dimensional computed tomography was used to investigate 101 pertrochanteric fractures treated with fixed angle sliding hip screw devices, with emphasis on the comminuted cortex. A comminuted fracture was defined as a fracture that had a third fracture fragment at the main fracture line.
Results:
There were 40 fractures without comminution and 61 with comminution. All 61 comminuted fractures had a comminuted posterior cortex, and 3 of 61 fractures also had comminution at the anterior cortex. The prevalence of cutting out of the implant from the femoral head was significantly higher in cases involving comminution at both the posterior and anterior cortices than in cases involving comminution only at the posterior cortex (66.7 % and 3.4 %, p < 0.0001).
Conclusion:
The posterior cortex was comminuted in 60.4% of pertrochanteric fractures and the anterior cortex in 3.0%. Intended impaction at the fracture site could not be obtained at any cortex in cases with comminution at both the anterior and posterior cortices; comminution at the anterior cortex may be a predictor of cutting out.
INTRODUCTION
Pertrochanteric fractures are of great interest because of their high rates of postoperative fatality and they represent a serious health resource issue [1-4]. Although surgical approaches for treatment have evolved greatly, satisfactory outcomes have not always been achieved [5].
Fixed angle sliding hip screw devices are the gold standard in surgical treatment of pertrochanteric fractures [5]. The central concept of these devices is to allow the proximal head neck fragment and distal shaft fragment to impact under control and achieve bone on bone stability [6, 7]. However, this concept fails if the intended impaction cannot be obtained because of comminution at the fracture site. Poole et al. mapped cortical thickness of the proximal femur using a novel computed tomography (CT) image processing technique [8]. The constructed images showed that the anterior cortex was thicker than the posterior cortex [8]. With regard to the incidence of fractures, the posterior cortex of the trochanteric region is empirically recognized to be frequently comminuted [7, 9, 10]. Thus, many investigators have recommended avoiding fracture reduction that could introduce impaction at the posterior cortex during surgical treatment of pertrochanteric fractures with fixed angle sliding hip screw devices [7, 9-13]. Nevertheless, the frequency of comminution at the posterior cortex remains unclear, and to our knowledge there have been no investigations of comminution at the anterior cortex. The anterior cortex was considered to perform a more important role in sustaining the sliding bone fragment [9, 10]. Thus, fracture types with comminuted anterior cortices were speculated to have greater instability.
Recent studies have highlighted the advantages of the availability of three-dimensional CT reconstructions for assessment of fractures [14-16]. We assessed comminution of pertrochanteric fractures using three-dimensional CT because conventional plain X-ray examination was considered to have limited accuracy. This study was performed to determine the frequency of comminution in pertrochanteric fractures. Specifically, we examined the following two hypotheses: (1) fractures with a comminuted cortex have greater risk of failure and (2) three-dimensional CT analysis can reveal the fracture pattern that is not recognized in the classification based on X-ray examination.
MATERIALS AND METHODS
The study protocol and publication of this retrospective study were approved by our institutional review board. The requirement for informed consent was waived based on the decision of our institutional review board because this retrospective study did not include any intervention or any personally identifiable information.
The criteria for inclusion were cases of AO/OTA 31-A1 or A2 pertrochanteric fractures that were treated with fixed angle sliding hip screw devices between December 2009 and March 2012 [17].
The following cases were excluded from the study: solitary greater trochanteric fracture, AO/OTA 31-A3 intertrochanteric fracture, occult fracture that could be diagnosed only by magnetic resonance imaging [18], history of a fracture or surgery involving the ipsilateral hip, pathological fractures (except severe osteoporosis), and patients who were considered by their attending physicians to not require three-dimensional CT assessment.
A fixed angle sliding hip screw device was used for internal fixation in all cases. Ninety six screw and side plate devices and five intramedullary devices were used to accomplish fixation. The selection criteria for the two implants were not specified in writing, with the final decision being made by the operating surgeon.
Physical therapy and sitting position were started on the first postoperative day. When treated with screw and side plate devices, the patients in whom weight bearing was considered unsuitable because of instability of the fracture site remained without weight bearing for two to three postoperative weeks. Full weight bearing was allowed on the first postoperative day for patients treated with intramedullary devices.
Radiographic Evaluation
The pertrochanteric fracture was assessed on three-dimensional CT reconstruction images before surgery. ECLOS software for CT (Hitachi Medical Corporation, Tokyo, Japan) was used to create the three-dimensional images. No additional CT scan was performed after surgery.
We retrospectively reviewed the images with emphasis on comminution of the fracture site. A comminuted fracture was defined as a fracture that had a third fracture fragment at the main fracture line. Fractures with a third fracture fragment at the anterior or posterior cortex were defined as having anterior or posterior comminution, respectively. However, third fragments involving only the tip of the greater trochanter were not considered to be comminution (Fig. 1).
Nakano used three-dimensional CT reconstruction images to study the pattern of trochanteric fractures, and established a classification based on the finding that all fracture patterns could be differentiated by assessing the combination of four parts: the head neck fragment, greater trochanter fragment, lesser trochanter fragment, and shaft fragment [19]. We modified the classification of Nakano and divided the comminutions of the posterior cortex into those with (A) a third fragment consisting only of the lesser trochanter Fig. (2-A), (B) a third fragment consisting only of the greater trochanter that was larger than the tip fracture Fig. (2-B), Fig. (2C) a third fragment consisting of the greater trochanter and lesser trochanter en bloc Fig. (2-C), and (D) a third fragment consisting of the greater trochanter and lesser trochanter separately (Fig. 2-D).
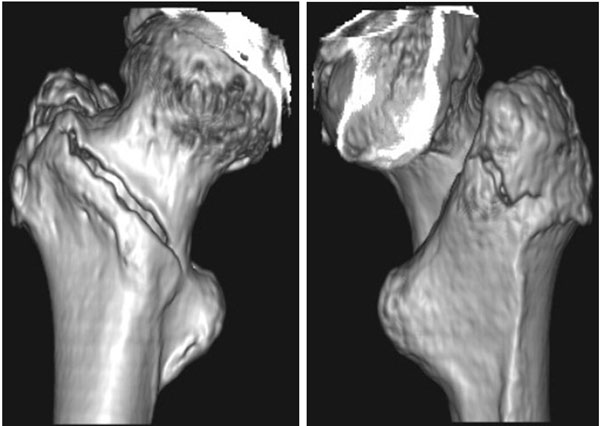
Pertrochanteric fracture with the third fragment consisting of only the tip of the greater trochanter. This pattern of fracture was not defined as comminuted.
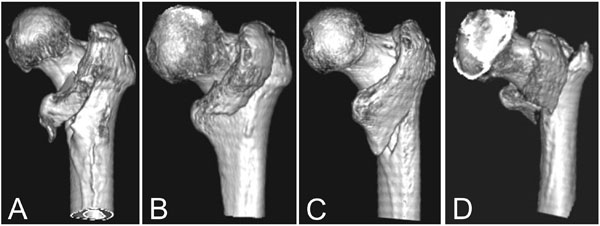
Four patterns of comminuted posterior cortex in pertrochanteric fractures. All three-dimensional reconstructed images were observed in the posteroanterior direction.
A) A third fragment consisting of the lesser trochanter.
B) A third fragment consisting of the relatively larger greater trochanter.
C) A third fragment consisting of the greater trochanter and lesser trochanter en bloc.
D) A third fragment consisting of the greater trochanter and lesser trochanter separately.
In the cases treated with a screw and side plate device, sliding distance was measured on anteroposterior radiographs [10, 20, 21]. The distance was defined as the distance the inserted lag screw slid within the fixed angle sliding hip screw device. To correct radiographic magnification and hip joint rotation, we used the ratio of the length of the lag screw measured on the radiograph to the length of the lag screw actually inserted (Fig. 3). We calculated the difference between immediately after surgery and at the time of final follow-up.
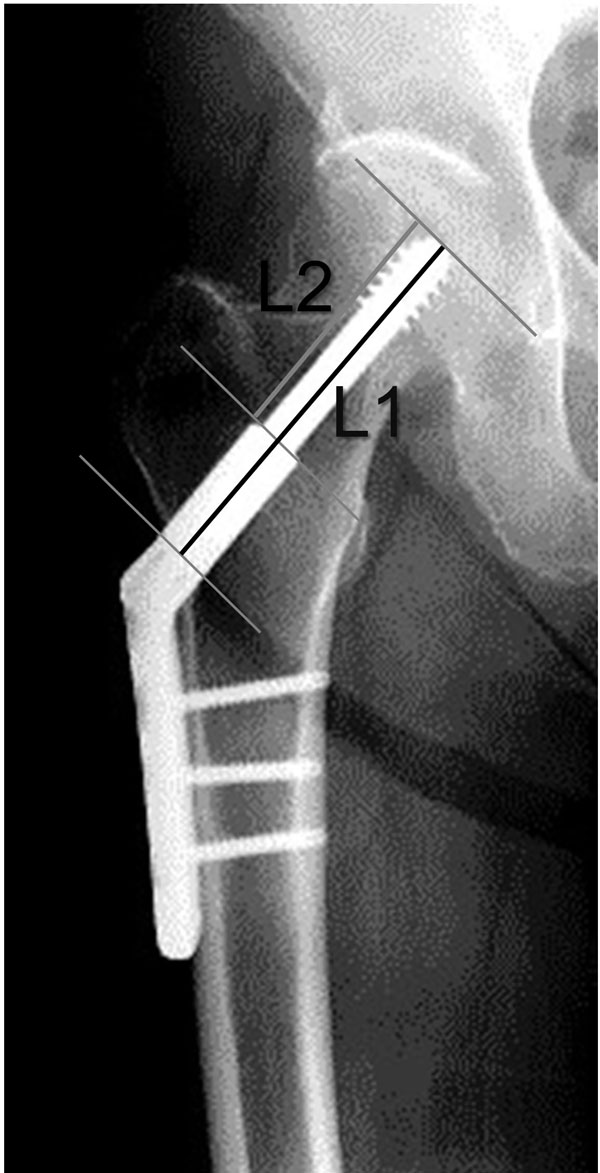
When measuring sliding distance, corrections were made using the ratio of screw length (L1) measured on the radiograph to actual screw length (L). The distance from the tip of the screw to the implant as measured on the radiograph was recorded as L2. The corrected distance from the tip of the screw to the implant was calculated using the following equation: L2 × (L / L1). The difference between corrected distance at final observation and corrected distance immediately after surgery was calculated as the sliding distance. The distance from the tip of the screw to the barrel was measured.
All radiographic evaluations were reassessed by an investigator (ST) who was not employed at the study institution during the study period.
Clinical Evaluation
We investigated the clinical outcomes to determine the incidence of cutting out of the implant from the femoral head as the endpoint following the recommendations of Baumgaertner et al. [22].
First, we compared the cutting out rates between the cases with and without comminution to assess the hypothesis of this study that fractures with comminution had higher risk of failure. Second, we compared the cutting out rate between the cases with comminution at the posterior cortex and those with comminution at the anterior cortex because the anterior cortex may introduce greater instability and poorer outcome. In addition, we compared patient demographics and baseline clinical characteristics, including sex, age, height, weight, implant type (screw and side plate device or intramedullary device), and tip apex distance between groups [22].
Statistical Analysis
One way analysis of variance was used to compare the sliding distance between fracture types.
To compare the cutting out rate between groups, the Mann-Whitney U test was used to analyze continuous data, whereas the χ2 test was used to analyze data grouped into distinct categories. For all analyses, the significance level was set at p < 0.05.
RESULTS
From the records of 125 consecutively treated fractures during the study period, 101 fractures met the inclusion criteria. Eight cases were excluded because CT evaluation was not performed before surgery. All cases that were not evaluated by CT were diagnosed by plain X-ray examination to be simple two part fractures. Moreover, 11 occult fractures and one fracture in a patient with a history of arthrodesis and AO/OTA 31-A3 fractures were also excluded. The mean ± standard deviation age, height, and weight of the 87 female and 14 male patients were 83.6 ± 8.2 years (range, 54-98 years), 148.1 ± 8.9 cm (range, 129-172 cm), and 45.1 ± 8.4 kg (range, 29-69 kg), respectively.
Radiographic Evaluation
There were 40 of 101 (39.6 %) fractures with no comminution. Of these 40, there were 22 (21.8 %) simple two part fractures and 18 (17.8 %) fractures involving the tip of the greater trochanter.
Sixty-one of the 101 fractures (60.4 %) had comminutions at the posterior cortex and three (3.0 %) had comminutions at the anterior cortex (Fig. 4). All of the fractures that had comminuted anterior cortices also exhibited comminution at the posterior cortices.
Of 61 fractures with comminution at the posterior cortex, four (4.0 %) had third fragments consisting of only the lesser trochanter, 23 (22.8 %) had third fragments consisting of the larger greater trochanter fragment, 17 (16.8 %) had third fragments consisting of the greater trochanter and lesser trochanter en bloc, and 17 (16.8 %) had third fragments consisting of the greater trochanter and lesser trochanter separately.
In all three fractures with both the anterior and posterior comminuted cortex, the fracture sites were shattered (Fig. 4).
The sliding distance was 5.2 ± 6.2 mm in fractures with no comminution, 5.1 ± 5.4 mm in fractures with a third fragment consisting of the large greater trochanter, 8.2 ± 3.2 mm fractures with a third fragment consisting of only the lesser trochanter, 5.5 ± 5.0 mm in fractures with third fragment consisting of the greater trochanter and lesser trochanter en bloc, and 9.1 ± 6.8 mm in fractures with a third fragment consisting of the greater trochanter and lesser trochanter separately. The sliding distance was not significantly different between groups (p = 0.13, one-way analysis of variance).
Clinical Evaluation
Cutting out of the implant from the femoral head occurred in four of the 101 fractures (4.0 %). The cases with no comminutions had no cutting out. All four cases of cutting out occurred in the fractures with comminution. Although the cutting out rate tended to be lower in fractures without than in those with comminution (0 % versus 6.6 %, respectively), the difference was not significant (p = 0.09, χ2 test). The preoperative characteristics are presented in Table 1. There were no differences in sex, age, height, weight, or tip apex distance between the two groups.
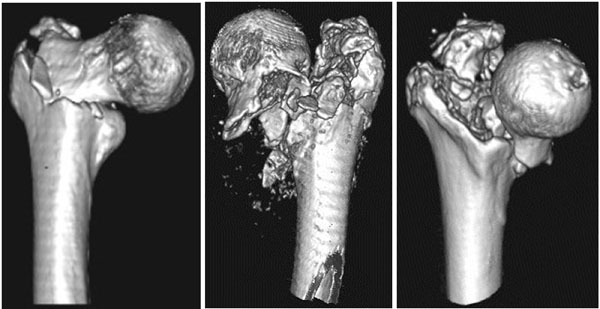
Three-dimensional reconstructed images in three cases of trochanteric fracture with comminuted anterior cortex. The three-dimensional reconstructed image was observed in the anteroposterior direction. Third fragments at the anterior fracture site were present. In all cases, the fragments did not shape mass, but were broken into small pieces.
Patient demographics and baseline clinical characteristics when comparing the clinical outcomes between fractures with and without comminution.
Fractures without comminution (n = 40) |
Fractures with comminution (n = 61) |
p-value | |
---|---|---|---|
Age, years | 85 (54 - 97) | 85 (65 - 98) | 0.72* |
Sex (F/M) | 34/6 | 53/8 | 0.79† |
Height, cm | 148 (136 - 172) | 149 (129 - 172) | 0.26* |
Weight, kg | 45 (30 - 67) | 45 (29 - 69) | 0.44* |
Implant type (plate/nail) | 40/0 | 56/5 | 0.59† |
Tip apex distance | 16.9 (9.1 - 33.1) | 18.7 (9.1 - 34.2) | 0.09* |
Cutting out occurred in two of 58 cases with comminutions at the posterior cortex alone (3.4 %). The cases with comminutions at both the anterior and posterior cortices showed cutting out in two of three cases (66.7 %), and had a significantly higher rate of cutting out than those with comminutions only at the posterior cortex (p < 0.0001, χ2 test). Excessive femoral shaft medialization occurred in the remaining one case with comminutions at both the anterior and posterior cortices; the extent of sliding distance along the lag screw was 21.5 mm when the fracture healed. The preoperative characteristics are presented in Table 2, and no differences were found in terms of sex, age, height, weight, or tip apex distance.
Patient demographics and baseline clinical characteristics when comparing the clinical outcomes between fractures with posterior cortex comminution and those with posterior and anterior cortex comminution.
Comminution only at the posterior cortex (n = 58) |
Comminution at both the anterior and posterior cortex (n = 3) |
p-value | |
---|---|---|---|
Age, years | 82 (54-98) | 83 (79-85) | 0.80* |
Sex (F/M) | 50/8 | 3/0 | 0.49† |
Height, cm | 149 (129-172) | 148 (144-151) | 0.88* |
Weight, kg | 45 (29-69) | 47 (44-50) | 0.71* |
Implant type (plate/nail) | 53/5 | 3/0 | 0.59† |
Tip apex distance | 19.1 (9.1-34.2) | 23.6 (10.1-30.0) | 0.61* |
DISCUSSION
This study showed that three-dimensional CT analysis could reveal fractures with a comminuted cortex had a larger risk of cutting out, and the fracture patterns that were not recognized in the classification based on X-ray examination.
Fixed angle sliding hip screw devices offer a biomechanical advantage by allowing the two major fragments to impact under control [6, 23]. Impaction will occur until the proximal bone rests on the distal bone fragment. However, if effective bone-on-bone impaction is not applied, excessive collapse and cutting out of the implant from the femoral head can occur [24]. The fracture pattern of a comminuted posterior cortex has been recognized as an unstable fracture type [25-27]. However, the rate of comminution had not been sufficiently clarified. In the present study, three-dimensional CT analysis showed that 60.4 % of cases of pertrochanteric fracture had comminution at the posterior cortex.
Internal fixation with fixed angle sliding hip screw devices for fractures with comminution at the posterior cortex could not introduce the intended impaction when the head neck fragment was displaced posterior to the shaft fragment [7, 9-13]. To resolve this issue, the head neck fragment should be displaced anterior to the shaft fragment [7, 9-13]. Nevertheless, if the comminution presents not only at the posterior cortex but also at the anterior cortex, controlled impaction can rarely be achieved at any of the cortices.
Three-dimensional CT analysis revealed that 16.8 % of the third fracture fragments involved the greater and lesser trochanters en bloc. This fracture type has not been demonstrated in the classification of pertrochanteric fractures based on plain X-ray examination [25-27]. In 2006, Nakano and his colleagues reported that the frequency of this fracture type was 18.2 % (17/93 trochanteric fractures) based on research using three-dimensional CT reconstructions [19, 28].
There were several limitations in our study. The principal limitation was its retrospective design, although there were no differences in sex, age, height, weight, or tip apex distance between the groups in comparison of cutting out rate.
The small number of fractures with comminution at both the anterior and posterior cortex was another important limitation. Although the cutting out rate was significant higher in this fracture type, the small sample size could have distorted the statistical conclusions. We believe that a comminuted anterior cortex may be an important predictor of cutting out. However, further studies with larger sample sizes are needed for rigorous analysis.
None of the fractures without comminution and 6.6 % of the fractures with comminution had cutting out of the implant from the femoral head, and there were no significant differences between the groups. Based on the difference in cutting out rate in our study, a sample size of 143 patients per treatment arm was calculated, a type I error rate of 5 %, and a type II error rate of 20 %. As our study was underpowered, we could not definitively conclude that the cutting out rate was not different between groups. We acknowledge these limitations, but believe that our findings will help improve treatment outcomes for pertrochanteric fractures.
The causes of cutting out are multifactorial, and include surgical technique, fracture pattern, fracture reduction, bone quality, and implant design [10, 22, 29, 30]. This study included the limitation that the bone quality of study patients was not measured quantitatively and two different implant designs were employed for internal fixation.
In conclusion, comminution of the posterior or anterior cortex was observed in 60.4 % and 3.0 % of the 101 pertrochanteric fractures studied, respectively. All cases with comminution of the anterior cortex also showed comminution at the posterior cortex, and may have a higher risk of cutting out.
CONFLICT OF INTEREST
The authors declare that they did not receive and will not receive any benefits or funding from any commercial party related directly or indirectly to the subject of this article.
ACKNOWLEDGMENTS
The authors sincerely thank Dr. Tetsuo Nakano for allowing us to refer to the classification for trochanteric fracture.